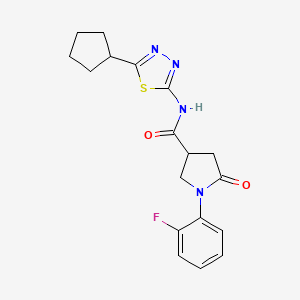
N-(5-cyclopentyl-1,3,4-thiadiazol-2-yl)-1-(2-fluorophenyl)-5-oxopyrrolidine-3-carboxamide
- Click on QUICK INQUIRY to receive a quote from our team of experts.
- With the quality product at a COMPETITIVE price, you can focus more on your research.
Overview
Description
N-(5-cyclopentyl-1,3,4-thiadiazol-2-yl)-1-(2-fluorophenyl)-5-oxopyrrolidine-3-carboxamide is a complex organic compound that has garnered interest in various scientific fields due to its unique chemical structure and potential applications. This compound features a thiadiazole ring, a fluorophenyl group, and a pyrrolidine carboxamide moiety, which contribute to its distinct chemical properties and reactivity.
Preparation Methods
Synthetic Routes and Reaction Conditions
The synthesis of N-(5-cyclopentyl-1,3,4-thiadiazol-2-yl)-1-(2-fluorophenyl)-5-oxopyrrolidine-3-carboxamide typically involves multiple steps, starting from readily available precursors. One common synthetic route includes the following steps:
Formation of the Thiadiazole Ring: This step involves the cyclization of appropriate precursors under specific conditions to form the 1,3,4-thiadiazole ring.
Introduction of the Fluorophenyl Group: The fluorophenyl group is introduced through a substitution reaction, often using a fluorinated benzene derivative.
Formation of the Pyrrolidine Carboxamide Moiety:
Industrial Production Methods
Industrial production of this compound may involve optimization of the synthetic route to ensure high yield and purity. Techniques such as continuous flow synthesis and the use of advanced catalysts can be employed to enhance the efficiency of the production process.
Chemical Reactions Analysis
Types of Reactions
N-(5-cyclopentyl-1,3,4-thiadiazol-2-yl)-1-(2-fluorophenyl)-5-oxopyrrolidine-3-carboxamide can undergo various chemical reactions, including:
Oxidation: This compound can be oxidized under specific conditions to form corresponding oxidized products.
Reduction: Reduction reactions can be performed to modify the functional groups within the compound.
Substitution: The compound can undergo substitution reactions, particularly at the fluorophenyl group, to introduce different substituents.
Common Reagents and Conditions
Oxidation: Common oxidizing agents such as potassium permanganate or hydrogen peroxide can be used.
Reduction: Reducing agents like sodium borohydride or lithium aluminum hydride are typically employed.
Substitution: Halogenated reagents and catalysts like palladium on carbon (Pd/C) can facilitate substitution reactions.
Major Products
The major products formed from these reactions depend on the specific conditions and reagents used. For example, oxidation may yield oxidized derivatives, while substitution can introduce various functional groups to the fluorophenyl ring.
Scientific Research Applications
N-(5-cyclopentyl-1,3,4-thiadiazol-2-yl)-1-(2-fluorophenyl)-5-oxopyrrolidine-3-carboxamide has a wide range of applications in scientific research:
Chemistry: It is used as a building block for the synthesis of more complex molecules and as a reagent in various chemical reactions.
Biology: The compound is studied for its potential biological activities, including antimicrobial and anticancer properties.
Medicine: Research is ongoing to explore its potential as a therapeutic agent for various diseases.
Industry: It is used in the development of new materials and as a catalyst in industrial processes.
Mechanism of Action
The mechanism of action of N-(5-cyclopentyl-1,3,4-thiadiazol-2-yl)-1-(2-fluorophenyl)-5-oxopyrrolidine-3-carboxamide involves its interaction with specific molecular targets and pathways. The compound may bind to enzymes or receptors, modulating their activity and leading to various biological effects. The exact molecular targets and pathways can vary depending on the specific application and context.
Comparison with Similar Compounds
Similar Compounds
- N-(5-cyclopentyl-1,3,4-thiadiazol-2-yl)-1-(2-chlorophenyl)-5-oxopyrrolidine-3-carboxamide
- N-(5-cyclopentyl-1,3,4-thiadiazol-2-yl)-1-(2-bromophenyl)-5-oxopyrrolidine-3-carboxamide
Uniqueness
N-(5-cyclopentyl-1,3,4-thiadiazol-2-yl)-1-(2-fluorophenyl)-5-oxopyrrolidine-3-carboxamide is unique due to the presence of the fluorophenyl group, which imparts distinct chemical properties and reactivity compared to its analogs with different halogen substituents
Properties
Molecular Formula |
C18H19FN4O2S |
---|---|
Molecular Weight |
374.4 g/mol |
IUPAC Name |
N-(5-cyclopentyl-1,3,4-thiadiazol-2-yl)-1-(2-fluorophenyl)-5-oxopyrrolidine-3-carboxamide |
InChI |
InChI=1S/C18H19FN4O2S/c19-13-7-3-4-8-14(13)23-10-12(9-15(23)24)16(25)20-18-22-21-17(26-18)11-5-1-2-6-11/h3-4,7-8,11-12H,1-2,5-6,9-10H2,(H,20,22,25) |
InChI Key |
HYYPSJZIPUFOKW-UHFFFAOYSA-N |
Canonical SMILES |
C1CCC(C1)C2=NN=C(S2)NC(=O)C3CC(=O)N(C3)C4=CC=CC=C4F |
Origin of Product |
United States |
Disclaimer and Information on In-Vitro Research Products
Please be aware that all articles and product information presented on BenchChem are intended solely for informational purposes. The products available for purchase on BenchChem are specifically designed for in-vitro studies, which are conducted outside of living organisms. In-vitro studies, derived from the Latin term "in glass," involve experiments performed in controlled laboratory settings using cells or tissues. It is important to note that these products are not categorized as medicines or drugs, and they have not received approval from the FDA for the prevention, treatment, or cure of any medical condition, ailment, or disease. We must emphasize that any form of bodily introduction of these products into humans or animals is strictly prohibited by law. It is essential to adhere to these guidelines to ensure compliance with legal and ethical standards in research and experimentation.