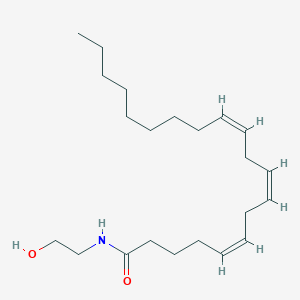
Mead acid ethanolamide
Overview
Description
Mead acid-EA is a N-acylethanolamine 20:3.
Mechanism of Action
Target of Action
Mead Acid-EA is an n-9 polyunsaturated fatty acid (PUFA) that is distributed in various normal tissues . It can be converted to several specific lipid mediators by lipoxygenase and cyclooxygenase . These enzymes are the primary targets of Mead Acid-EA, playing a crucial role in its physiological and pathological effects.
Mode of Action
Mead Acid-EA interacts with its targets, lipoxygenase and cyclooxygenase, to produce specific lipid mediators . These mediators then induce changes in the body, affecting various physiological processes. The exact nature of these changes is still under investigation, but they are believed to have significant effects on inflammation, cancer, dermatitis, and cystic fibrosis .
Biochemical Pathways
Mead Acid-EA affects the biochemical pathways of PUFA metabolism . It is synthesized from oleic acid in the n-9 series via the same enzyme system as for the n-6 and n-3 series . This synthesis process impacts the levels of other PUFAs in the body, influencing various physiological roles and diseases .
Pharmacokinetics
It is known that mead acid-ea is distributed in various normal tissues , suggesting a wide distribution in the body
Result of Action
The action of Mead Acid-EA results in the production of specific lipid mediators, which have various effects on the body . These effects include potential impacts on inflammation, cancer, dermatitis, and cystic fibrosis . .
Action Environment
The action, efficacy, and stability of Mead Acid-EA can be influenced by various environmental factors. For instance, essential fatty acid (EFA) deficiency can lead to an increase in the synthesis of Mead Acid-EA . This suggests that dietary factors and overall health status can significantly influence the action of Mead Acid-EA.
Biochemical Analysis
Biochemical Properties
Mead Acid-EA plays an important role in biochemical reactions. It interacts with enzymes such as lipoxygenase and cyclooxygenase, which convert it into specific lipid mediators . These interactions are crucial for its function as an endogenous multifunctional PUFA .
Cellular Effects
Mead Acid-EA has been found to have effects on inflammation, cancer, dermatitis, and cystic fibrosis . It influences cell function by altering the production of inflammatory mediators, modulating neutrophils infiltration, and altering VEGF effector pathway .
Molecular Mechanism
The molecular mechanism of Mead Acid-EA involves its conversion to specific lipid mediators by lipoxygenase and cyclooxygenase . This process allows it to exert its effects at the molecular level, including binding interactions with biomolecules, enzyme inhibition or activation, and changes in gene expression .
Temporal Effects in Laboratory Settings
It is known that it is widely distributed in various normal tissues , suggesting that it may have long-term effects on cellular function.
Metabolic Pathways
Mead Acid-EA is involved in the metabolism of PUFAs . It is synthesized from oleic acid in the n-9 series via the same enzyme system as for the n-6 and n-3 series . It may also have effects on metabolic flux or metabolite levels.
Transport and Distribution
Mead Acid-EA is transported and distributed within cells and tissues. The absorption and distribution of Mead Acid-EA is widespread physiologically and is similar to that of other C20 PUFAs, such as ARA and EPA .
Properties
IUPAC Name |
(5Z,8Z,11Z)-N-(2-hydroxyethyl)icosa-5,8,11-trienamide | |
---|---|---|
Source | PubChem | |
URL | https://pubchem.ncbi.nlm.nih.gov | |
Description | Data deposited in or computed by PubChem | |
InChI |
InChI=1S/C22H39NO2/c1-2-3-4-5-6-7-8-9-10-11-12-13-14-15-16-17-18-19-22(25)23-20-21-24/h9-10,12-13,15-16,24H,2-8,11,14,17-21H2,1H3,(H,23,25)/b10-9-,13-12-,16-15- | |
Source | PubChem | |
URL | https://pubchem.ncbi.nlm.nih.gov | |
Description | Data deposited in or computed by PubChem | |
InChI Key |
YKGQBEGMUSSPFY-YOILPLPUSA-N | |
Source | PubChem | |
URL | https://pubchem.ncbi.nlm.nih.gov | |
Description | Data deposited in or computed by PubChem | |
Canonical SMILES |
CCCCCCCCC=CCC=CCC=CCCCC(=O)NCCO | |
Source | PubChem | |
URL | https://pubchem.ncbi.nlm.nih.gov | |
Description | Data deposited in or computed by PubChem | |
Isomeric SMILES |
CCCCCCCC/C=C\C/C=C\C/C=C\CCCC(=O)NCCO | |
Source | PubChem | |
URL | https://pubchem.ncbi.nlm.nih.gov | |
Description | Data deposited in or computed by PubChem | |
Molecular Formula |
C22H39NO2 | |
Source | PubChem | |
URL | https://pubchem.ncbi.nlm.nih.gov | |
Description | Data deposited in or computed by PubChem | |
DSSTOX Substance ID |
DTXSID901348030 | |
Record name | (5Z,8Z,11Z)-N-(2-Hydroxyethyl)-5,8,11-icosatrienamide | |
Source | EPA DSSTox | |
URL | https://comptox.epa.gov/dashboard/DTXSID901348030 | |
Description | DSSTox provides a high quality public chemistry resource for supporting improved predictive toxicology. | |
Molecular Weight |
349.5 g/mol | |
Source | PubChem | |
URL | https://pubchem.ncbi.nlm.nih.gov | |
Description | Data deposited in or computed by PubChem | |
CAS No. |
169232-04-6 | |
Record name | (5Z,8Z,11Z)-N-(2-Hydroxyethyl)-5,8,11-icosatrienamide | |
Source | EPA DSSTox | |
URL | https://comptox.epa.gov/dashboard/DTXSID901348030 | |
Description | DSSTox provides a high quality public chemistry resource for supporting improved predictive toxicology. | |
Retrosynthesis Analysis
AI-Powered Synthesis Planning: Our tool employs the Template_relevance Pistachio, Template_relevance Bkms_metabolic, Template_relevance Pistachio_ringbreaker, Template_relevance Reaxys, Template_relevance Reaxys_biocatalysis model, leveraging a vast database of chemical reactions to predict feasible synthetic routes.
One-Step Synthesis Focus: Specifically designed for one-step synthesis, it provides concise and direct routes for your target compounds, streamlining the synthesis process.
Accurate Predictions: Utilizing the extensive PISTACHIO, BKMS_METABOLIC, PISTACHIO_RINGBREAKER, REAXYS, REAXYS_BIOCATALYSIS database, our tool offers high-accuracy predictions, reflecting the latest in chemical research and data.
Strategy Settings
Precursor scoring | Relevance Heuristic |
---|---|
Min. plausibility | 0.01 |
Model | Template_relevance |
Template Set | Pistachio/Bkms_metabolic/Pistachio_ringbreaker/Reaxys/Reaxys_biocatalysis |
Top-N result to add to graph | 6 |
Feasible Synthetic Routes
Q1: How does MEAD ethanolamide, also known as N-(5Z,8Z,11Z-eicosatrienoyl)-ethanolamine, interact with its target and what are the downstream effects?
A1: MEAD ethanolamide acts as an agonist at both cannabinoid receptor subtypes, CB1 and CB2. [, , ] This means it binds to these receptors and activates them, mimicking the effects of endogenous cannabinoids like anandamide.
Q2: What is known about the synthesis of MEAD ethanolamide in biological systems?
A3: Research suggests that MEAD ethanolamide can be synthesized enzymatically in a similar manner to anandamide. [] This involves the condensation of mead acid with ethanolamine. Studies using rat and human hippocampal P2 membranes demonstrated that both mead acid and arachidonic acid were equally effective substrates for the enzymatic synthesis of their respective ethanolamides. [] This finding suggests a potential parallel pathway for the biosynthesis of these endocannabinoids, possibly responding to specific physiological conditions or dietary factors.
Disclaimer and Information on In-Vitro Research Products
Please be aware that all articles and product information presented on BenchChem are intended solely for informational purposes. The products available for purchase on BenchChem are specifically designed for in-vitro studies, which are conducted outside of living organisms. In-vitro studies, derived from the Latin term "in glass," involve experiments performed in controlled laboratory settings using cells or tissues. It is important to note that these products are not categorized as medicines or drugs, and they have not received approval from the FDA for the prevention, treatment, or cure of any medical condition, ailment, or disease. We must emphasize that any form of bodily introduction of these products into humans or animals is strictly prohibited by law. It is essential to adhere to these guidelines to ensure compliance with legal and ethical standards in research and experimentation.