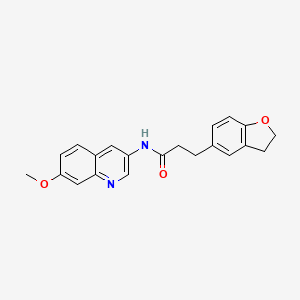
3-(2,3-dihydro-1-benzofuran-5-yl)-N-(7-methoxyquinolin-3-yl)propanamide
- Click on QUICK INQUIRY to receive a quote from our team of experts.
- With the quality product at a COMPETITIVE price, you can focus more on your research.
Overview
Description
3-(2,3-dihydro-1-benzofuran-5-yl)-N-(7-methoxyquinolin-3-yl)propanamide is a synthetic organic compound that belongs to the class of amides
Preparation Methods
Synthetic Routes and Reaction Conditions
The synthesis of 3-(2,3-dihydro-1-benzofuran-5-yl)-N-(7-methoxyquinolin-3-yl)propanamide typically involves multi-step organic reactions. A common synthetic route might include:
Formation of the Benzofuran Ring: Starting from a suitable phenol derivative, the benzofuran ring can be synthesized through cyclization reactions.
Quinoline Ring Synthesis: The quinoline ring can be synthesized from aniline derivatives through various methods, such as the Skraup synthesis.
Amide Bond Formation: The final step involves coupling the benzofuran and quinoline derivatives with a propanamide group using reagents like carbodiimides or coupling agents like EDCI (1-ethyl-3-(3-dimethylaminopropyl)carbodiimide).
Industrial Production Methods
Industrial production of this compound would likely involve optimization of the above synthetic routes to ensure high yield and purity. This might include the use of continuous flow reactors and advanced purification techniques like chromatography.
Chemical Reactions Analysis
Types of Reactions
3-(2,3-dihydro-1-benzofuran-5-yl)-N-(7-methoxyquinolin-3-yl)propanamide can undergo various chemical reactions, including:
Oxidation: The compound can be oxidized using reagents like potassium permanganate or chromium trioxide.
Reduction: Reduction reactions can be carried out using hydrogen gas in the presence of a catalyst like palladium on carbon.
Substitution: The compound can undergo nucleophilic or electrophilic substitution reactions, depending on the functional groups present.
Common Reagents and Conditions
Oxidation: Potassium permanganate in acidic or basic conditions.
Reduction: Hydrogen gas with palladium on carbon.
Substitution: Halogenating agents like N-bromosuccinimide for electrophilic substitution.
Major Products Formed
The major products formed from these reactions would depend on the specific conditions and reagents used. For example, oxidation might yield carboxylic acids, while reduction could produce alcohols or amines.
Scientific Research Applications
Chemistry: As an intermediate in the synthesis of more complex molecules.
Biology: Potential use as a probe or ligand in biochemical assays.
Medicine: Possible applications in drug discovery and development, particularly in targeting specific enzymes or receptors.
Industry: Use in the production of specialty chemicals or materials.
Mechanism of Action
The mechanism of action of 3-(2,3-dihydro-1-benzofuran-5-yl)-N-(7-methoxyquinolin-3-yl)propanamide would depend on its specific interactions with molecular targets. It might act by binding to specific proteins or enzymes, thereby modulating their activity. The pathways involved could include signal transduction, metabolic pathways, or gene expression regulation.
Comparison with Similar Compounds
Similar Compounds
3-(2,3-dihydro-1-benzofuran-5-yl)-N-(quinolin-3-yl)propanamide: Lacks the methoxy group on the quinoline ring.
3-(benzofuran-5-yl)-N-(7-methoxyquinolin-3-yl)propanamide: Lacks the dihydro group on the benzofuran ring.
Uniqueness
The presence of both the benzofuran and quinoline rings, along with the specific substitution pattern, makes 3-(2,3-dihydro-1-benzofuran-5-yl)-N-(7-methoxyquinolin-3-yl)propanamide unique. This unique structure could confer specific biological activities or chemical reactivity that distinguishes it from similar compounds.
Properties
Molecular Formula |
C21H20N2O3 |
---|---|
Molecular Weight |
348.4 g/mol |
IUPAC Name |
3-(2,3-dihydro-1-benzofuran-5-yl)-N-(7-methoxyquinolin-3-yl)propanamide |
InChI |
InChI=1S/C21H20N2O3/c1-25-18-5-4-15-11-17(13-22-19(15)12-18)23-21(24)7-3-14-2-6-20-16(10-14)8-9-26-20/h2,4-6,10-13H,3,7-9H2,1H3,(H,23,24) |
InChI Key |
HQSGEJMYAUXYIS-UHFFFAOYSA-N |
Canonical SMILES |
COC1=CC2=NC=C(C=C2C=C1)NC(=O)CCC3=CC4=C(C=C3)OCC4 |
Origin of Product |
United States |
Disclaimer and Information on In-Vitro Research Products
Please be aware that all articles and product information presented on BenchChem are intended solely for informational purposes. The products available for purchase on BenchChem are specifically designed for in-vitro studies, which are conducted outside of living organisms. In-vitro studies, derived from the Latin term "in glass," involve experiments performed in controlled laboratory settings using cells or tissues. It is important to note that these products are not categorized as medicines or drugs, and they have not received approval from the FDA for the prevention, treatment, or cure of any medical condition, ailment, or disease. We must emphasize that any form of bodily introduction of these products into humans or animals is strictly prohibited by law. It is essential to adhere to these guidelines to ensure compliance with legal and ethical standards in research and experimentation.