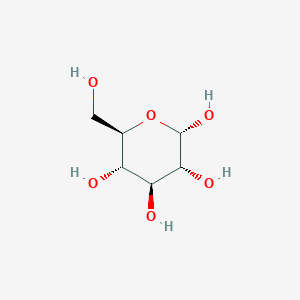
alpha-D-glucose
Overview
Description
Alpha-D-glucose is a monosaccharide, a simple sugar that is a fundamental building block of carbohydrates. It is one of the two anomers of D-glucose, the other being beta-D-glucose. The difference between these two forms lies in the orientation of the hydroxyl group on the first carbon atom. This compound is a crucial energy source for living organisms and plays a significant role in various metabolic processes.
Mechanism of Action
Target of Action
Alpha-D-Glucose, also known as Glucopyranose, alpha-D-, alpha-Dextrose, alpha-glucose, or alpha-D-Glucopyranose, primarily targets several enzymes. These include Hexokinase-1, Phosphomannomutase/phosphoglucomutase, Maltose-6’-phosphate glucosidase, Glycogen phosphorylase, muscle form, Alpha,alpha-trehalose-phosphate synthase [UDP-forming], Glutamine–fructose-6-phosphate aminotransferase [isomerizing], 6-phospho-beta-glucosidase BglT, Glucose-6-phosphate isomerase, and Aldose reductase . These enzymes play crucial roles in various metabolic processes, including glycolysis/gluconeogenesis pathway .
Mode of Action
This compound acts as an inhibitor of alpha-glucosidase, an enzyme that catalyzes the hydrolysis of alpha bonds of large, alpha-linked polysaccharides, such as starch and glycogen . By inhibiting these enzymes, this compound limits the absorption of dietary carbohydrates and the subsequent postprandial increase in blood glucose and insulin levels .
Biochemical Pathways
This compound is an essential metabolite in virtually all organisms as it participates in a variety of biological processes. It is an intermediate in the glycolysis/gluconeogenesis pathway . It is also involved in the tricarboxylic acid (TCA) cycle, a central metabolic network in most organisms .
Pharmacokinetics
It is known that this compound inhibitors retard glucose absorption, which can have implications for bioavailability .
Result of Action
The primary result of this compound’s action is the reduction of postprandial blood glucose and insulin levels. This is achieved by inhibiting the activity of alpha-glucosidase enzymes, which limits the absorption of dietary carbohydrates . This can be beneficial in managing conditions like type 2 diabetes .
Action Environment
The action of this compound can be influenced by various environmental factors. For instance, the efficacy of this compound as an alpha-glucosidase inhibitor can be affected by the presence of other compounds in the environment . Additionally, the stability of this compound can be influenced by factors such as pH and temperature .
Biochemical Analysis
Biochemical Properties
Alpha-D-Glucose participates in numerous biochemical reactions. It is the substrate for the enzyme alpha-glucosidase, which catalyzes the liberation of alpha-glucose from nonreducing ends of alpha-glucosides or from complex polymers with alpha- (1-4) bonds . This enzyme has been found to have transglucosylase activity, producing isomalto- and malto-oligosaccharides .
Molecular Mechanism
The molecular mechanism of this compound involves its interaction with various biomolecules. It binds to specific enzymes, leading to enzyme activation or inhibition, and induces changes in gene expression. For instance, in the presence of this compound, the enzyme alpha-glucosidase undergoes an auto-phosphorylation step, which is essential for its functionality .
Temporal Effects in Laboratory Settings
In laboratory settings, the effects of this compound can change over time. It has been observed that the enzyme alpha-glucosidase from Aspergillus niger ITV-01, which uses this compound as a substrate, has an optimum temperature of 80°C but is stable at 40°C . This indicates the stability of this compound and its long-term effects on cellular function.
Preparation Methods
Synthetic Routes and Reaction Conditions: Alpha-D-glucose can be synthesized through the acetylation of D-glucose, followed by anomerization of the beta-anomer with acetyl anhydride catalyzed by Lewis acids . This method involves treating beta-D-glucose pentaacetate with Lewis acids to obtain the alpha form, which is a classic anomerization method .
Industrial Production Methods: In industrial settings, this compound is often produced through the enzymatic hydrolysis of starch. Starch, a polysaccharide composed of glucose units, is broken down by enzymes such as amylase and glucosidase to yield glucose monomers, including this compound .
Chemical Reactions Analysis
Types of Reactions: Alpha-D-glucose undergoes various chemical reactions, including oxidation, reduction, and glycosylation.
Common Reagents and Conditions:
Reduction: It can be reduced to sorbitol using reducing agents such as sodium borohydride.
Major Products:
Oxidation: Gluconic acid
Reduction: Sorbitol
Glycosylation: Glycosides
Scientific Research Applications
Alpha-D-glucose has a wide range of applications in scientific research:
Biology: this compound is essential in studying cellular respiration and energy metabolism.
Industry: It is used in the production of biofuels and biodegradable plastics.
Comparison with Similar Compounds
Beta-D-glucose: Another anomer of D-glucose with a different orientation of the hydroxyl group on the first carbon.
Fructose: A ketohexose that is structurally different but also a simple sugar.
Galactose: An aldohexose similar to glucose but with a different arrangement of hydroxyl groups.
Alpha-D-glucose’s unique structure and reactivity make it a vital compound in various biological and industrial processes.
Properties
Glucose supplies most of the energy to all tissues by generating energy molecules ATP and NADH during a series of metabolism reactions called glycolysis. Glycolysis can be divided into two main phases where the preparatory phase is initiated by the phosphorylation of glucose by hexokinase to form glucose 6-phosphate. The addition of the high-energy phosphate group activates glucose for the subsequent breakdown in later steps of glycolysis and is the rate-limiting step. Products end up as substrates for following reactions, to ultimately convert C6 glucose molecule into two C3 sugar molecules. These products enter the energy-releasing phase where the total of 4ATP and 2NADH molecules are generated per one glucose molecule. The total aerobic metabolism of glucose can produce up to 36 ATP molecules. These energy-producing reactions of glucose are limited to D-glucose as L-glucose cannot be phosphorylated by hexokinase. Glucose can act as precursors to generate other biomolecules such as vitamin C. It plays a role as a signaling molecule to control glucose and energy homeostasis. Glucose can regulate gene transcription, enzyme activity, hormone secretion, and the activity of glucoregulatory neurons. The types, number, and kinetics of glucose transporters expressed depends on the tissues and fine-tunes glucose uptake, metabolism, and signal generation to preserve cellular and whole body metabolic integrity. | |
CAS No. |
492-62-6 |
Molecular Formula |
C6H12O6 |
Molecular Weight |
180.16 g/mol |
IUPAC Name |
(2S,3S,4S,5S,6S)-6-(hydroxymethyl)oxane-2,3,4,5-tetrol |
InChI |
InChI=1S/C6H12O6/c7-1-2-3(8)4(9)5(10)6(11)12-2/h2-11H,1H2/t2-,3+,4-,5-,6-/m0/s1 |
InChI Key |
WQZGKKKJIJFFOK-GNFDWLABSA-N |
Isomeric SMILES |
C([C@H]1[C@H]([C@@H]([C@@H]([C@H](O1)O)O)O)O)O |
SMILES |
C(C1C(C(C(C(O1)O)O)O)O)O |
Canonical SMILES |
C(C1C(C(C(C(O1)O)O)O)O)O |
Appearance |
Powder |
density |
Relative density (water = 1): 1.56 |
melting_point |
133.0 °C 146°C 146 °C |
492-62-6 26655-34-5 50-99-7 |
|
physical_description |
Dry Powder; Liquid, Other Solid; NKRA; Liquid White crystalline powder; Odorless; [Acros Organics MSDS] |
solubility |
Soluble |
Synonyms |
α-D-Glucose; α-Dextrose; α-Glucose; |
vapor_pressure |
8.02e-14 mmHg |
Origin of Product |
United States |
Synthesis routes and methods I
Procedure details
Synthesis routes and methods II
Procedure details
Retrosynthesis Analysis
AI-Powered Synthesis Planning: Our tool employs the Template_relevance Pistachio, Template_relevance Bkms_metabolic, Template_relevance Pistachio_ringbreaker, Template_relevance Reaxys, Template_relevance Reaxys_biocatalysis model, leveraging a vast database of chemical reactions to predict feasible synthetic routes.
One-Step Synthesis Focus: Specifically designed for one-step synthesis, it provides concise and direct routes for your target compounds, streamlining the synthesis process.
Accurate Predictions: Utilizing the extensive PISTACHIO, BKMS_METABOLIC, PISTACHIO_RINGBREAKER, REAXYS, REAXYS_BIOCATALYSIS database, our tool offers high-accuracy predictions, reflecting the latest in chemical research and data.
Strategy Settings
Precursor scoring | Relevance Heuristic |
---|---|
Min. plausibility | 0.01 |
Model | Template_relevance |
Template Set | Pistachio/Bkms_metabolic/Pistachio_ringbreaker/Reaxys/Reaxys_biocatalysis |
Top-N result to add to graph | 6 |
Feasible Synthetic Routes
Q1: How does the anomeric form of D-glucose influence its interaction with hexokinases?
A1: Research indicates that both yeast and bovine hexokinases exhibit anomeric specificity, albeit in opposite directions. At 30°C, yeast hexokinase demonstrates a higher maximal velocity and affinity for alpha-D-glucose compared to beta-D-glucose. Conversely, bovine hexokinase shows a preference for beta-D-glucose in terms of maximal velocity while exhibiting higher affinity for this compound. Interestingly, these anomeric preferences are temperature-dependent. []
Q2: Does the anomeric specificity of glucose phosphorylation always reflect the anomeric preference of the phosphorylating enzyme?
A2: Not necessarily. Studies on rat erythrocyte homogenates, which lack glucokinase and utilize a beta-D-glucose preferring hexokinase, reveal a higher glycolytic rate with this compound. This suggests that factors beyond enzyme specificity influence the anomeric preference of glycolysis in intact cells. []
Q3: Does this compound pentaacetate affect glycogen synthase activity in hepatocytes?
A3: Yes, this compound pentaacetate has been shown to enhance glycogen synthase a activity in rat hepatocytes. This suggests a potential role for this compound in promoting glycogen synthesis, which could be relevant for managing blood glucose levels. []
Q4: How does this compound interact with Mycoplasma fermentans?
A4: Research suggests that this compound acts as a receptor for Mycoplasma fermentans adhesion to human epithelial cells, although the affinity is low. This interaction is proposed to play a role in the pathogenicity of this bacterium. []
Q5: What is the molecular formula and weight of this compound?
A5: The molecular formula of this compound is C6H12O6, and its molecular weight is 180.16 g/mol.
Q6: How can vibrational spectroscopy contribute to the structural analysis of this compound?
A6: Matrix-isolation FT-IR spectroscopy combined with DFT calculations allows for the identification and assignment of vibrational modes in this compound. This technique enables the characterization of different conformers and provides insights into the hydrogen bonding network within the molecule. []
Q7: Can IR spectroscopy be used for quantitative analysis of this compound in ionic liquids?
A7: Yes, IR spectroscopy has been successfully employed for quantitative analysis of this compound in the ionic liquid 1-ethyl-3-methylimidazolium acetate ([EMIM][OAc]). This approach provides a convenient method for monitoring sugar concentrations in ILs and studying the kinetics of enzymatic reactions in these solvents. []
Q8: How does the anomeric form of D-glucose affect its role as a substrate for maltose phosphorylase?
A8: Maltose phosphorylase exhibits strict specificity for this compound as a cosubstrate in reactions with beta-D-glucosyl fluoride, leading to the formation of alpha-maltose. This specificity stems from the essential interaction between the axial 1-OH group of this compound and a specific protein group within the enzyme's active site. []
Q9: Can trehalase utilize non-glycosidic substrates, and what is the role of this compound in these reactions?
A9: Yes, trehalase can utilize beta-D-glucosyl fluoride as a substrate in the presence of specific aldopyranose acceptors. This compound is uniquely effective in promoting the enzymatic release of fluoride from beta-D-glucosyl fluoride, highlighting its crucial role as a cosubstrate in these reactions. []
Q10: How can molecular modeling contribute to understanding the inhibition of glycogen phosphorylase by glucose analogues?
A10: Molecular docking and dynamics simulations provide valuable insights into the binding modes of glucose analogues to glycogen phosphorylase b. These techniques help rationalize the observed affinities and guide the design of more potent inhibitors with potential antihyperglycemic properties. [, ]
Q11: How do structural modifications at the C1 position of glucose influence its inhibitory activity against glycogen phosphorylase?
A11: Studies on various glucose analogues reveal that the presence and orientation of substituents at the C1 position significantly impact their inhibitory potency against glycogen phosphorylase b. Alpha-linked substituents generally lead to better inhibition compared to beta-linked modifications. Additionally, analogues with rigid substituents tend to exhibit greater inhibitory activity than those with flexible groups. []
Q12: What is the role of the glucokinase regulatory protein in modulating the anomeric specificity of glucokinase?
A12: The glucokinase regulatory protein, in the presence of D-fructose 6-phosphate, differentially affects the phosphorylation of alpha- and beta-D-glucose by glucokinase. Liver glucokinase exhibits greater resistance to this inhibitory action when phosphorylating this compound compared to beta-D-glucose. While a similar trend is observed with B-cell glucokinase, it does not reach statistical significance. []
Q13: Can this compound influence the transport of other molecules in biological systems?
A13: Research indicates that this compound can inhibit the transport of L-ascorbic acid in cultured bovine retinal capillary pericytes. This inhibition is proposed to occur through a shared facilitated carrier diffusion system, suggesting a potential interaction between glucose and ascorbate transport mechanisms in these cells. []
Disclaimer and Information on In-Vitro Research Products
Please be aware that all articles and product information presented on BenchChem are intended solely for informational purposes. The products available for purchase on BenchChem are specifically designed for in-vitro studies, which are conducted outside of living organisms. In-vitro studies, derived from the Latin term "in glass," involve experiments performed in controlled laboratory settings using cells or tissues. It is important to note that these products are not categorized as medicines or drugs, and they have not received approval from the FDA for the prevention, treatment, or cure of any medical condition, ailment, or disease. We must emphasize that any form of bodily introduction of these products into humans or animals is strictly prohibited by law. It is essential to adhere to these guidelines to ensure compliance with legal and ethical standards in research and experimentation.