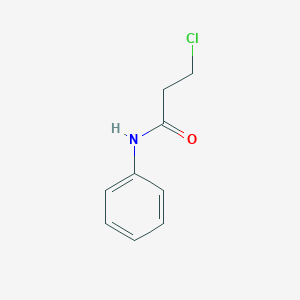
3-Chloro-N-phenylpropanamide
Overview
Description
3-Chloro-N-phenylpropanamide is an organic compound with the molecular formula C9H10ClNO. It is a white solid that is widely used in the pharmaceutical and chemical industries. This compound is an important intermediate in the synthesis of various compounds, including pharmaceutical drugs and agrochemicals .
Mechanism of Action
Biochemical Pathways
It’s known that this compound can be used in the synthesis of functional acrylamides, which are valuable intermediates for organic synthesis and building blocks in many industrial areas, like polymer synthesis, fine chemicals, and pharmaceutics .
Pharmacokinetics
As a synthetic intermediate, it’s primarily used in laboratory settings rather than administered in biological systems .
Result of Action
As a synthetic intermediate, its primary role is in the synthesis of other compounds rather than exerting a direct biological effect .
Action Environment
The action of 3-Chloro-N-phenylpropanamide is influenced by various environmental factors. For instance, its reactivity can be affected by temperature, pH, and the presence of other chemicals. In a laboratory setting, the reaction conditions can be carefully controlled to optimize the yield of the desired product .
Preparation Methods
Synthetic Routes and Reaction Conditions
3-Chloro-N-phenylpropanamide can be synthesized through the Schotten-Baumann reaction, which involves the reaction of aniline with 3-chloropropanoyl chloride in the presence of a base such as sodium bicarbonate . The reaction is typically carried out at room temperature using an aprotic solvent like toluene .
Industrial Production Methods
In industrial settings, the synthesis of this compound can be achieved through a continuous flow process. This method allows for the safe and on-demand synthesis of the compound with high yield. The continuous flow process minimizes side-products and enables a safe and convenient scale-up .
Chemical Reactions Analysis
Types of Reactions
3-Chloro-N-phenylpropanamide undergoes various chemical reactions, including:
Substitution Reactions: The chlorine atom can be substituted with other functional groups.
Reduction Reactions: The compound can be reduced to form amines.
Elimination Reactions: The compound can undergo thermal elimination to form acrylamides.
Common Reagents and Conditions
Substitution Reactions: Reagents such as sodium hydroxide or potassium hydroxide can be used.
Reduction Reactions: Reducing agents like lithium aluminum hydride or sodium borohydride are commonly used.
Elimination Reactions: The reaction is typically carried out at elevated temperatures (around 100°C) in the presence of a base like potassium hydroxide.
Major Products Formed
Substitution Reactions: Various substituted phenylpropanamides.
Reduction Reactions: Amines.
Elimination Reactions: Acrylamides.
Scientific Research Applications
3-Chloro-N-phenylpropanamide has several scientific research applications, including:
Chemistry: It is used as an intermediate in the synthesis of various organic compounds.
Biology: The compound is used in the study of biochemical pathways and enzyme reactions.
Medicine: It serves as a precursor in the synthesis of pharmaceutical drugs.
Industry: The compound is used in the production of agrochemicals and other industrial chemicals.
Comparison with Similar Compounds
Similar Compounds
- N-phenylpropanamide
- 3-Chloropropanamide
- N-phenylacrylamide
Uniqueness
3-Chloro-N-phenylpropanamide is unique due to the presence of both a phenyl group and a chlorine atom in its structure. This combination imparts specific chemical properties, making it a valuable intermediate in organic synthesis. The compound’s ability to undergo various chemical reactions, such as substitution and elimination, further enhances its versatility in scientific research and industrial applications .
Properties
IUPAC Name |
3-chloro-N-phenylpropanamide | |
---|---|---|
Source | PubChem | |
URL | https://pubchem.ncbi.nlm.nih.gov | |
Description | Data deposited in or computed by PubChem | |
InChI |
InChI=1S/C9H10ClNO/c10-7-6-9(12)11-8-4-2-1-3-5-8/h1-5H,6-7H2,(H,11,12) | |
Source | PubChem | |
URL | https://pubchem.ncbi.nlm.nih.gov | |
Description | Data deposited in or computed by PubChem | |
InChI Key |
FRXJYUFHAXXSAL-UHFFFAOYSA-N | |
Source | PubChem | |
URL | https://pubchem.ncbi.nlm.nih.gov | |
Description | Data deposited in or computed by PubChem | |
Canonical SMILES |
C1=CC=C(C=C1)NC(=O)CCCl | |
Source | PubChem | |
URL | https://pubchem.ncbi.nlm.nih.gov | |
Description | Data deposited in or computed by PubChem | |
Molecular Formula |
C9H10ClNO | |
Source | PubChem | |
URL | https://pubchem.ncbi.nlm.nih.gov | |
Description | Data deposited in or computed by PubChem | |
DSSTOX Substance ID |
DTXSID40286583 | |
Record name | 3-Chloro-N-phenylpropanamide | |
Source | EPA DSSTox | |
URL | https://comptox.epa.gov/dashboard/DTXSID40286583 | |
Description | DSSTox provides a high quality public chemistry resource for supporting improved predictive toxicology. | |
Molecular Weight |
183.63 g/mol | |
Source | PubChem | |
URL | https://pubchem.ncbi.nlm.nih.gov | |
Description | Data deposited in or computed by PubChem | |
CAS No. |
3460-04-6 | |
Record name | 3460-04-6 | |
Source | DTP/NCI | |
URL | https://dtp.cancer.gov/dtpstandard/servlet/dwindex?searchtype=NSC&outputformat=html&searchlist=46582 | |
Description | The NCI Development Therapeutics Program (DTP) provides services and resources to the academic and private-sector research communities worldwide to facilitate the discovery and development of new cancer therapeutic agents. | |
Explanation | Unless otherwise indicated, all text within NCI products is free of copyright and may be reused without our permission. Credit the National Cancer Institute as the source. | |
Record name | 3-Chloro-N-phenylpropanamide | |
Source | EPA DSSTox | |
URL | https://comptox.epa.gov/dashboard/DTXSID40286583 | |
Description | DSSTox provides a high quality public chemistry resource for supporting improved predictive toxicology. | |
Synthesis routes and methods I
Procedure details
Synthesis routes and methods II
Procedure details
Synthesis routes and methods III
Procedure details
Retrosynthesis Analysis
AI-Powered Synthesis Planning: Our tool employs the Template_relevance Pistachio, Template_relevance Bkms_metabolic, Template_relevance Pistachio_ringbreaker, Template_relevance Reaxys, Template_relevance Reaxys_biocatalysis model, leveraging a vast database of chemical reactions to predict feasible synthetic routes.
One-Step Synthesis Focus: Specifically designed for one-step synthesis, it provides concise and direct routes for your target compounds, streamlining the synthesis process.
Accurate Predictions: Utilizing the extensive PISTACHIO, BKMS_METABOLIC, PISTACHIO_RINGBREAKER, REAXYS, REAXYS_BIOCATALYSIS database, our tool offers high-accuracy predictions, reflecting the latest in chemical research and data.
Strategy Settings
Precursor scoring | Relevance Heuristic |
---|---|
Min. plausibility | 0.01 |
Model | Template_relevance |
Template Set | Pistachio/Bkms_metabolic/Pistachio_ringbreaker/Reaxys/Reaxys_biocatalysis |
Top-N result to add to graph | 6 |
Feasible Synthetic Routes
Disclaimer and Information on In-Vitro Research Products
Please be aware that all articles and product information presented on BenchChem are intended solely for informational purposes. The products available for purchase on BenchChem are specifically designed for in-vitro studies, which are conducted outside of living organisms. In-vitro studies, derived from the Latin term "in glass," involve experiments performed in controlled laboratory settings using cells or tissues. It is important to note that these products are not categorized as medicines or drugs, and they have not received approval from the FDA for the prevention, treatment, or cure of any medical condition, ailment, or disease. We must emphasize that any form of bodily introduction of these products into humans or animals is strictly prohibited by law. It is essential to adhere to these guidelines to ensure compliance with legal and ethical standards in research and experimentation.