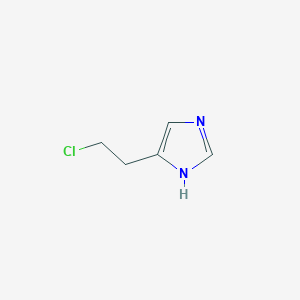
4-(2-chloroethyl)-1H-imidazole
Overview
Description
4-(2-chloroethyl)-1H-imidazole is an organic compound that belongs to the class of imidazoles. Imidazoles are heterocyclic compounds containing a five-membered ring with two non-adjacent nitrogen atoms. The presence of the 2-chloroethyl group in the 4-position of the imidazole ring imparts unique chemical properties to this compound, making it of interest in various fields of research and industry.
Preparation Methods
Synthetic Routes and Reaction Conditions
The synthesis of 4-(2-chloroethyl)-1H-imidazole typically involves the alkylation of imidazole with 2-chloroethyl chloride. The reaction is usually carried out in the presence of a base such as potassium carbonate or sodium hydroxide to facilitate the nucleophilic substitution reaction. The general reaction scheme is as follows:
Imidazole+2-chloroethyl chloride→this compound
The reaction is typically conducted in an organic solvent such as dimethylformamide (DMF) or dimethyl sulfoxide (DMSO) at elevated temperatures to ensure complete conversion.
Industrial Production Methods
On an industrial scale, the production of this compound may involve continuous flow processes to enhance efficiency and yield. The use of automated reactors and optimized reaction conditions can significantly reduce production costs and improve the purity of the final product.
Chemical Reactions Analysis
Types of Reactions
4-(2-chloroethyl)-1H-imidazole can undergo various chemical reactions, including:
Nucleophilic Substitution: The chloroethyl group can be replaced by other nucleophiles such as amines, thiols, or alkoxides.
Oxidation: The imidazole ring can be oxidized to form imidazole N-oxides.
Reduction: The compound can be reduced to form 4-(2-hydroxyethyl)-1H-imidazole.
Common Reagents and Conditions
Nucleophilic Substitution: Reagents such as sodium azide, potassium thiolate, or sodium alkoxide in polar aprotic solvents like DMF or DMSO.
Oxidation: Oxidizing agents such as hydrogen peroxide or m-chloroperbenzoic acid.
Reduction: Reducing agents such as lithium aluminum hydride or sodium borohydride.
Major Products Formed
Nucleophilic Substitution: Formation of substituted imidazoles with various functional groups.
Oxidation: Formation of imidazole N-oxides.
Reduction: Formation of 4-(2-hydroxyethyl)-1H-imidazole.
Scientific Research Applications
4-(2-chloroethyl)-1H-imidazole has several applications in scientific research, including:
Chemistry: Used as a building block for the synthesis of more complex molecules and as a reagent in organic synthesis.
Biology: Investigated for its potential biological activities, including antimicrobial and anticancer properties.
Medicine: Explored as a potential therapeutic agent due to its ability to interact with biological targets.
Industry: Utilized in the production of pharmaceuticals, agrochemicals, and other specialty chemicals.
Mechanism of Action
The mechanism of action of 4-(2-chloroethyl)-1H-imidazole involves its ability to alkylate nucleophilic sites in biological molecules. The 2-chloroethyl group can form covalent bonds with nucleophilic centers such as the nitrogen atoms in DNA bases, leading to the formation of cross-links and inhibition of DNA replication. This property makes it a potential candidate for anticancer therapy, as it can induce cell death in rapidly dividing cancer cells.
Comparison with Similar Compounds
Similar Compounds
4-(2-chloroethyl)morpholine: Another compound with a 2-chloroethyl group, but with a morpholine ring instead of an imidazole ring.
Mustard Gas (bis(2-chloroethyl) sulfide): A well-known alkylating agent with two 2-chloroethyl groups attached to a sulfur atom.
Uniqueness
4-(2-chloroethyl)-1H-imidazole is unique due to the presence of the imidazole ring, which imparts distinct chemical and biological properties. Unlike mustard gas, which is highly toxic and used as a chemical weapon, this compound is primarily of interest for its potential therapeutic applications and its use as a versatile building block in organic synthesis.
Properties
IUPAC Name |
5-(2-chloroethyl)-1H-imidazole | |
---|---|---|
Source | PubChem | |
URL | https://pubchem.ncbi.nlm.nih.gov | |
Description | Data deposited in or computed by PubChem | |
InChI |
InChI=1S/C5H7ClN2/c6-2-1-5-3-7-4-8-5/h3-4H,1-2H2,(H,7,8) | |
Source | PubChem | |
URL | https://pubchem.ncbi.nlm.nih.gov | |
Description | Data deposited in or computed by PubChem | |
InChI Key |
LJBSKUABXVDRGS-UHFFFAOYSA-N | |
Source | PubChem | |
URL | https://pubchem.ncbi.nlm.nih.gov | |
Description | Data deposited in or computed by PubChem | |
Canonical SMILES |
C1=C(NC=N1)CCCl | |
Source | PubChem | |
URL | https://pubchem.ncbi.nlm.nih.gov | |
Description | Data deposited in or computed by PubChem | |
Molecular Formula |
C5H7ClN2 | |
Source | PubChem | |
URL | https://pubchem.ncbi.nlm.nih.gov | |
Description | Data deposited in or computed by PubChem | |
DSSTOX Substance ID |
DTXSID80330256 | |
Record name | 4-(2-chloroethyl)-1H-imidazole | |
Source | EPA DSSTox | |
URL | https://comptox.epa.gov/dashboard/DTXSID80330256 | |
Description | DSSTox provides a high quality public chemistry resource for supporting improved predictive toxicology. | |
Molecular Weight |
130.57 g/mol | |
Source | PubChem | |
URL | https://pubchem.ncbi.nlm.nih.gov | |
Description | Data deposited in or computed by PubChem | |
CAS No. |
13518-55-3 | |
Record name | 4-(2-chloroethyl)-1H-imidazole | |
Source | EPA DSSTox | |
URL | https://comptox.epa.gov/dashboard/DTXSID80330256 | |
Description | DSSTox provides a high quality public chemistry resource for supporting improved predictive toxicology. | |
Retrosynthesis Analysis
AI-Powered Synthesis Planning: Our tool employs the Template_relevance Pistachio, Template_relevance Bkms_metabolic, Template_relevance Pistachio_ringbreaker, Template_relevance Reaxys, Template_relevance Reaxys_biocatalysis model, leveraging a vast database of chemical reactions to predict feasible synthetic routes.
One-Step Synthesis Focus: Specifically designed for one-step synthesis, it provides concise and direct routes for your target compounds, streamlining the synthesis process.
Accurate Predictions: Utilizing the extensive PISTACHIO, BKMS_METABOLIC, PISTACHIO_RINGBREAKER, REAXYS, REAXYS_BIOCATALYSIS database, our tool offers high-accuracy predictions, reflecting the latest in chemical research and data.
Strategy Settings
Precursor scoring | Relevance Heuristic |
---|---|
Min. plausibility | 0.01 |
Model | Template_relevance |
Template Set | Pistachio/Bkms_metabolic/Pistachio_ringbreaker/Reaxys/Reaxys_biocatalysis |
Top-N result to add to graph | 6 |
Feasible Synthetic Routes
Disclaimer and Information on In-Vitro Research Products
Please be aware that all articles and product information presented on BenchChem are intended solely for informational purposes. The products available for purchase on BenchChem are specifically designed for in-vitro studies, which are conducted outside of living organisms. In-vitro studies, derived from the Latin term "in glass," involve experiments performed in controlled laboratory settings using cells or tissues. It is important to note that these products are not categorized as medicines or drugs, and they have not received approval from the FDA for the prevention, treatment, or cure of any medical condition, ailment, or disease. We must emphasize that any form of bodily introduction of these products into humans or animals is strictly prohibited by law. It is essential to adhere to these guidelines to ensure compliance with legal and ethical standards in research and experimentation.