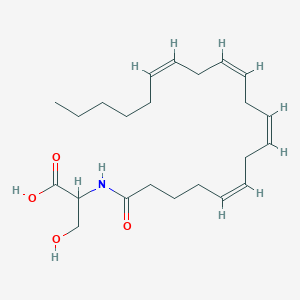
N-Arachidonoyl-L-Serine
Overview
Description
N-Arachidonoyl-L-Serine is a naturally occurring lipoamino acid found in the central nervous system. It is chemically related to N-arachidonoyl ethanolamine (anandamide), an endocannabinoid. This compound has been identified as an endocannabinoid-like compound with various physiological roles, including vasodilation and neuroprotection .
Mechanism of Action
Target of Action
N-Arachidonoyl-L-Serine (ARA-S) is a lipid molecule structurally related to the endocannabinoid family . It has been found to affect n-type ca2+ channels in rat sympathetic ganglion neurons .
Mode of Action
ARA-S’s interaction with its targets results in a rapid and reversible augmentation of Ca2+ current that is voltage-dependent and results from a hyperpolarizing shift in the activation curve . It appears to act independently of G-protein-coupled receptors .
Biochemical Pathways
ARA-S has been found to modulate Rho kinase, playing a critical role in the regulation of its activity and subsequent effects on the cytoskeleton . It also increases the phosphorylation of Akt and mitogen-activated protein kinase (MAPK) in human umbilical vein endothelial cells (HUVEC) . These effects are mediated via CB1, CB2, and TRPV1 receptors .
Pharmacokinetics
It is known to be an endogenous lipid found in the brain , suggesting that it can cross the blood-brain barrier
Result of Action
ARA-S has been shown to have neuroprotective effects . It leads to a significant improvement in functional outcome, reduced edema, and lesion volume following injury . It also induces relaxation in isolated rat mesenteric arteries and abdominal aorta . Moreover, it has been found to alter the cytoskeleton, supporting essential cell functions like vasodilation, proliferation, and movement .
Action Environment
The action of ARA-S can be influenced by various environmental factors. For instance, its effects on N-type Ca2+ channels were determined using whole cell patch clamp, a technique that allows for the control of the cell’s membrane potential . The precise mechanisms of action by ARA-S in various vascular preparations appear to be different and require further investigation .
Biochemical Analysis
Biochemical Properties
N-Arachidonoyl-L-Serine does not bind to central cannabinoid (CB1) and peripheral cannabinoid (CB2) receptors or vanilloid receptor 1 (VR1) . It has been found to modulate Rho kinase, which plays a critical role in the regulation of cytoskeletal activity and supports essential cell functions like vasodilation, proliferation, and movement .
Cellular Effects
This compound has been shown to have various effects on cells. It has been found to produce a rapid and reversible augmentation of Ca2+ current in rat sympathetic ganglion neurons, which was voltage-dependent and resulted from a hyperpolarizing shift in the activation curve . It also increases the proliferation of neural progenitor cells (NPCs) in vitro in cannabinoid-receptor-mediated mechanisms and maintains NPC in an undifferentiated state in vitro and in vivo .
Molecular Mechanism
It appears to act independently of G-protein-coupled receptors . It has been suggested that this compound may act via a novel, but as yet unidentified, G-protein-coupled non-CB1/CB2 cannabinoid receptor .
Preparation Methods
Synthetic Routes and Reaction Conditions: N-Arachidonoyl-L-Serine can be synthesized through the condensation of arachidonic acid with L-serine. The reaction typically involves the activation of the carboxyl group of arachidonic acid, followed by its coupling with the amino group of L-serine. Common reagents used in this process include dicyclohexylcarbodiimide (DCC) and N-hydroxysuccinimide (NHS) to facilitate the formation of the amide bond .
Industrial Production Methods:
Chemical Reactions Analysis
Types of Reactions: N-Arachidonoyl-L-Serine undergoes various chemical reactions, including:
Oxidation: It can be oxidized to form hydroperoxides and other oxygenated derivatives.
Reduction: Reduction reactions can convert it to more saturated analogs.
Substitution: this compound can participate in nucleophilic substitution reactions, where the serine moiety can be modified.
Common Reagents and Conditions:
Oxidation: Reagents such as hydrogen peroxide or molecular oxygen in the presence of catalysts.
Reduction: Catalytic hydrogenation using palladium on carbon (Pd/C) or other hydrogenation catalysts.
Substitution: Nucleophiles like amines or thiols under mild conditions.
Major Products:
Oxidation: Hydroperoxides and hydroxylated derivatives.
Reduction: Saturated analogs of this compound.
Substitution: Modified serine derivatives with various functional groups.
Scientific Research Applications
N-Arachidonoyl-L-Serine has a wide range of scientific research applications:
Chemistry: It serves as a model compound for studying amide bond formation and lipid biochemistry.
Biology: It is used to investigate the role of endocannabinoid-like compounds in cellular signaling and metabolism.
Medicine: this compound has potential therapeutic applications due to its vasodilatory and neuroprotective properties. It is being studied for its effects on cardiovascular health and neurodegenerative diseases.
Industry: Its unique properties make it a candidate for developing new pharmaceuticals and nutraceuticals
Comparison with Similar Compounds
N-Arachidonoyl-L-Serine is unique among endocannabinoid-like compounds due to its specific physiological effects and minimal activity at traditional cannabinoid receptors (CB1 and CB2). Similar compounds include:
N-Arachidonoyl Ethanolamine (Anandamide): A well-known endocannabinoid with significant activity at CB1 and CB2 receptors.
2-Arachidonoyl Glycerol (2-AG): Another endocannabinoid with similar receptor activity.
N-Arachidonoyl Dopamine: A compound with both endocannabinoid and dopaminergic activity
This compound stands out due to its unique receptor-independent mechanisms and potential therapeutic applications.
Properties
IUPAC Name |
(2S)-3-hydroxy-2-[[(5Z,8Z,11Z,14Z)-icosa-5,8,11,14-tetraenoyl]amino]propanoic acid | |
---|---|---|
Source | PubChem | |
URL | https://pubchem.ncbi.nlm.nih.gov | |
Description | Data deposited in or computed by PubChem | |
InChI |
InChI=1S/C23H37NO4/c1-2-3-4-5-6-7-8-9-10-11-12-13-14-15-16-17-18-19-22(26)24-21(20-25)23(27)28/h6-7,9-10,12-13,15-16,21,25H,2-5,8,11,14,17-20H2,1H3,(H,24,26)(H,27,28)/b7-6-,10-9-,13-12-,16-15-/t21-/m0/s1 | |
Source | PubChem | |
URL | https://pubchem.ncbi.nlm.nih.gov | |
Description | Data deposited in or computed by PubChem | |
InChI Key |
FQUVPTVNRMUOPO-UPQKDGGNSA-N | |
Source | PubChem | |
URL | https://pubchem.ncbi.nlm.nih.gov | |
Description | Data deposited in or computed by PubChem | |
Canonical SMILES |
CCCCCC=CCC=CCC=CCC=CCCCC(=O)NC(CO)C(=O)O | |
Source | PubChem | |
URL | https://pubchem.ncbi.nlm.nih.gov | |
Description | Data deposited in or computed by PubChem | |
Isomeric SMILES |
CCCCC/C=C\C/C=C\C/C=C\C/C=C\CCCC(=O)N[C@@H](CO)C(=O)O | |
Source | PubChem | |
URL | https://pubchem.ncbi.nlm.nih.gov | |
Description | Data deposited in or computed by PubChem | |
Molecular Formula |
C23H37NO4 | |
Source | PubChem | |
URL | https://pubchem.ncbi.nlm.nih.gov | |
Description | Data deposited in or computed by PubChem | |
Molecular Weight |
391.5 g/mol | |
Source | PubChem | |
URL | https://pubchem.ncbi.nlm.nih.gov | |
Description | Data deposited in or computed by PubChem | |
Retrosynthesis Analysis
AI-Powered Synthesis Planning: Our tool employs the Template_relevance Pistachio, Template_relevance Bkms_metabolic, Template_relevance Pistachio_ringbreaker, Template_relevance Reaxys, Template_relevance Reaxys_biocatalysis model, leveraging a vast database of chemical reactions to predict feasible synthetic routes.
One-Step Synthesis Focus: Specifically designed for one-step synthesis, it provides concise and direct routes for your target compounds, streamlining the synthesis process.
Accurate Predictions: Utilizing the extensive PISTACHIO, BKMS_METABOLIC, PISTACHIO_RINGBREAKER, REAXYS, REAXYS_BIOCATALYSIS database, our tool offers high-accuracy predictions, reflecting the latest in chemical research and data.
Strategy Settings
Precursor scoring | Relevance Heuristic |
---|---|
Min. plausibility | 0.01 |
Model | Template_relevance |
Template Set | Pistachio/Bkms_metabolic/Pistachio_ringbreaker/Reaxys/Reaxys_biocatalysis |
Top-N result to add to graph | 6 |
Feasible Synthetic Routes
Disclaimer and Information on In-Vitro Research Products
Please be aware that all articles and product information presented on BenchChem are intended solely for informational purposes. The products available for purchase on BenchChem are specifically designed for in-vitro studies, which are conducted outside of living organisms. In-vitro studies, derived from the Latin term "in glass," involve experiments performed in controlled laboratory settings using cells or tissues. It is important to note that these products are not categorized as medicines or drugs, and they have not received approval from the FDA for the prevention, treatment, or cure of any medical condition, ailment, or disease. We must emphasize that any form of bodily introduction of these products into humans or animals is strictly prohibited by law. It is essential to adhere to these guidelines to ensure compliance with legal and ethical standards in research and experimentation.