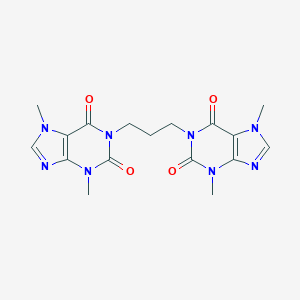
Bisdionin C
Overview
Description
Bisdionin C is a synthetic compound known for its potent inhibitory effects on chitinases, specifically acidic mammalian chitinase and chitotriosidase. These enzymes are involved in the hydrolysis of chitin, a polysaccharide found in the exoskeletons of arthropods and the cell walls of fungi.
Mechanism of Action
Target of Action
Bisdionin C is a potent inhibitor of GH18 chitinases . Its primary targets are acidic mammalian chitinase (AMCase) and chitotriosidase (CHIT1) , which are enzymes that play key roles in immunity, nutrition, pathogenicity, and arthropod molting . This compound also potently inhibits bacterial AfChiB1 chitinase .
Mode of Action
This compound interacts with its targets through competitive inhibition . A crystallographic structure of a chitinase-Bisdionin C complex shows the two aromatic systems of the ligand interacting with two conserved tryptophan residues exposed in the active site cleft of the enzyme, while at the same time forming extensive hydrogen-bonding interactions with the catalytic machinery .
Biochemical Pathways
This compound affects the biochemical pathways involving chitinases. Chitinases are glycosyl hydrolases that hydrolyze the β- (1-4)-linkage of N-acetyl-D-glucosamine units present in chitin polymers . By inhibiting chitinases, this compound can impact the degradation of chitin present in the cell wall of pathogens, potentially affecting the immune response .
Pharmacokinetics
It is described as a cell-permeable compound , which suggests it can cross cell membranes and therefore may have good bioavailability.
Result of Action
The inhibition of chitinases by this compound could potentially affect the body’s defense against chitin-containing pathogens . .
Biochemical Analysis
Biochemical Properties
Bisdionin C plays a significant role in biochemical reactions by inhibiting the activity of chitinases, including AMCase and CHIT1 . These enzymes are involved in the degradation of chitin, a polymer found in the cell walls of various organisms . This compound interacts with these enzymes, inhibiting their activity and thereby affecting the breakdown of chitin .
Cellular Effects
The inhibition of chitinases by this compound can have various effects on cells. As chitinases play a role in the immune response against chitin-containing pathogens, the inhibition of these enzymes by this compound could potentially affect this immune response .
Molecular Mechanism
This compound exerts its effects at the molecular level through its interactions with chitinases. It acts as a competitive inhibitor, binding to the active sites of these enzymes and preventing them from interacting with their substrate, chitin .
Metabolic Pathways
This compound, as an inhibitor of chitinases, could potentially affect the metabolic pathways involving these enzymes. Chitinases are involved in the breakdown of chitin, and their inhibition by this compound could affect the levels of chitin and its metabolites .
Preparation Methods
Synthetic Routes and Reaction Conditions
Bisdionin C is synthesized through a series of chemical reactions that involve the formation of a chitinase-inhibitor complex. The synthetic route typically begins with the preparation of a dicaffeine scaffold, which is then modified to introduce specific functional groups that enhance its inhibitory activity. The key steps in the synthesis include:
- Formation of the dicaffeine scaffold.
- Introduction of aromatic systems to interact with conserved tryptophan residues in the enzyme’s active site.
- Formation of hydrogen-bonding interactions with the catalytic machinery of the enzyme .
Industrial Production Methods
Industrial production of this compound involves scaling up the synthetic route to produce the compound in larger quantities. This process requires optimization of reaction conditions, such as temperature, pressure, and solvent choice, to ensure high yield and purity. The compound is typically produced as a powder and stored at low temperatures to maintain its stability .
Chemical Reactions Analysis
Types of Reactions
Bisdionin C primarily undergoes inhibition reactions with chitinases. It forms a complex with the enzyme, preventing it from hydrolyzing chitin. The compound does not undergo significant oxidation, reduction, or substitution reactions under normal conditions .
Common Reagents and Conditions
The synthesis of this compound involves reagents such as caffeine derivatives, aromatic compounds, and solvents like dimethyl sulfoxide. The reactions are typically carried out under controlled temperature and pressure conditions to ensure the formation of the desired product .
Major Products Formed
The major product formed from the synthesis of this compound is the chitinase-inhibitor complex. This complex is highly stable and exhibits potent inhibitory activity against chitinases .
Scientific Research Applications
Bisdionin C has a wide range of scientific research applications, including:
Chemistry: Used as a tool to study the structure and function of chitinases and to develop new chitinase inhibitors
Biology: Employed in research on the role of chitinases in various biological processes, such as immune response and pathogen defense
Medicine: Investigated for its potential therapeutic applications in treating diseases related to chitinase activity, such as asthma and allergic inflammation
Industry: Utilized in the development of new biocides and antifungal agents
Comparison with Similar Compounds
Bisdionin C is unique in its potent inhibitory activity and selectivity for chitinases. Similar compounds include:
Bisdionin F: Another chitinase inhibitor with a similar mechanism of action but different selectivity profile
Cipamfylline: A chitinase inhibitor with a different chemical structure and lower potency.
Istradefylline: A chitinase inhibitor used in research but with different pharmacological properties.
This compound stands out due to its high potency, selectivity, and stability, making it a valuable tool in scientific research and potential therapeutic applications .
Properties
IUPAC Name |
1-[3-(3,7-dimethyl-2,6-dioxopurin-1-yl)propyl]-3,7-dimethylpurine-2,6-dione | |
---|---|---|
Source | PubChem | |
URL | https://pubchem.ncbi.nlm.nih.gov | |
Description | Data deposited in or computed by PubChem | |
InChI |
InChI=1S/C17H20N8O4/c1-20-8-18-12-10(20)14(26)24(16(28)22(12)3)6-5-7-25-15(27)11-13(19-9-21(11)2)23(4)17(25)29/h8-9H,5-7H2,1-4H3 | |
Source | PubChem | |
URL | https://pubchem.ncbi.nlm.nih.gov | |
Description | Data deposited in or computed by PubChem | |
InChI Key |
KEPIKAFUZRKZMT-UHFFFAOYSA-N | |
Source | PubChem | |
URL | https://pubchem.ncbi.nlm.nih.gov | |
Description | Data deposited in or computed by PubChem | |
Canonical SMILES |
CN1C=NC2=C1C(=O)N(C(=O)N2C)CCCN3C(=O)C4=C(N=CN4C)N(C3=O)C | |
Source | PubChem | |
URL | https://pubchem.ncbi.nlm.nih.gov | |
Description | Data deposited in or computed by PubChem | |
Molecular Formula |
C17H20N8O4 | |
Source | PubChem | |
URL | https://pubchem.ncbi.nlm.nih.gov | |
Description | Data deposited in or computed by PubChem | |
Molecular Weight |
400.4 g/mol | |
Source | PubChem | |
URL | https://pubchem.ncbi.nlm.nih.gov | |
Description | Data deposited in or computed by PubChem | |
CAS No. |
74857-22-0 | |
Record name | 1,1-(Propane-1,3-diyl)bis(3,7-dimethyl-3,7-dihydro-1H-purine-2,6-dione) | |
Source | ChemIDplus | |
URL | https://pubchem.ncbi.nlm.nih.gov/substance/?source=chemidplus&sourceid=0074857220 | |
Description | ChemIDplus is a free, web search system that provides access to the structure and nomenclature authority files used for the identification of chemical substances cited in National Library of Medicine (NLM) databases, including the TOXNET system. | |
Record name | 1,1-(PROPANE-1,3-DIYL)BIS(3,7-DIMETHYL-3,7-DIHYDRO-1H-PURINE-2,6-DIONE) | |
Source | FDA Global Substance Registration System (GSRS) | |
URL | https://gsrs.ncats.nih.gov/ginas/app/beta/substances/WT3HN8U2ZZ | |
Description | The FDA Global Substance Registration System (GSRS) enables the efficient and accurate exchange of information on what substances are in regulated products. Instead of relying on names, which vary across regulatory domains, countries, and regions, the GSRS knowledge base makes it possible for substances to be defined by standardized, scientific descriptions. | |
Explanation | Unless otherwise noted, the contents of the FDA website (www.fda.gov), both text and graphics, are not copyrighted. They are in the public domain and may be republished, reprinted and otherwise used freely by anyone without the need to obtain permission from FDA. Credit to the U.S. Food and Drug Administration as the source is appreciated but not required. | |
Retrosynthesis Analysis
AI-Powered Synthesis Planning: Our tool employs the Template_relevance Pistachio, Template_relevance Bkms_metabolic, Template_relevance Pistachio_ringbreaker, Template_relevance Reaxys, Template_relevance Reaxys_biocatalysis model, leveraging a vast database of chemical reactions to predict feasible synthetic routes.
One-Step Synthesis Focus: Specifically designed for one-step synthesis, it provides concise and direct routes for your target compounds, streamlining the synthesis process.
Accurate Predictions: Utilizing the extensive PISTACHIO, BKMS_METABOLIC, PISTACHIO_RINGBREAKER, REAXYS, REAXYS_BIOCATALYSIS database, our tool offers high-accuracy predictions, reflecting the latest in chemical research and data.
Strategy Settings
Precursor scoring | Relevance Heuristic |
---|---|
Min. plausibility | 0.01 |
Model | Template_relevance |
Template Set | Pistachio/Bkms_metabolic/Pistachio_ringbreaker/Reaxys/Reaxys_biocatalysis |
Top-N result to add to graph | 6 |
Feasible Synthetic Routes
Q1: How does Bisdionin C interact with its target enzyme and what are the downstream effects of this interaction?
A1: this compound exhibits submicromolar inhibition of GH18 chitinases. [] Structural analysis reveals that this compound binds to the active site cleft of the enzyme, with its two aromatic rings forming interactions with two conserved tryptophan residues. [] Additionally, this compound establishes extensive hydrogen bonds with the catalytic machinery of the enzyme. [] This binding disrupts the enzyme's ability to break down chitin, a key component of fungal cell walls and some invertebrates' exoskeletons. Inhibiting chitinases in pathogenic organisms like fungi could potentially lead to their growth inhibition or death.
Q2: The research mentions that this compound shows selectivity for bacterial-type GH18 chitinases. What makes it a promising starting point for developing specific inhibitors, and are there any structural insights into this selectivity?
A2: this compound's binding mode, specifically its interactions with the conserved tryptophan residues and catalytic machinery within the active site cleft, suggests it could be a starting point for specific inhibitor development. [] While the provided research doesn't delve into the precise structural basis for its selectivity towards bacterial-type GH18 chitinases over plant-type ones, it implies that subtle differences in the active site architecture between these enzyme subtypes might be responsible. Further research exploring these structural differences and how this compound interacts with them is needed to confirm and understand the basis of this selectivity.
Disclaimer and Information on In-Vitro Research Products
Please be aware that all articles and product information presented on BenchChem are intended solely for informational purposes. The products available for purchase on BenchChem are specifically designed for in-vitro studies, which are conducted outside of living organisms. In-vitro studies, derived from the Latin term "in glass," involve experiments performed in controlled laboratory settings using cells or tissues. It is important to note that these products are not categorized as medicines or drugs, and they have not received approval from the FDA for the prevention, treatment, or cure of any medical condition, ailment, or disease. We must emphasize that any form of bodily introduction of these products into humans or animals is strictly prohibited by law. It is essential to adhere to these guidelines to ensure compliance with legal and ethical standards in research and experimentation.