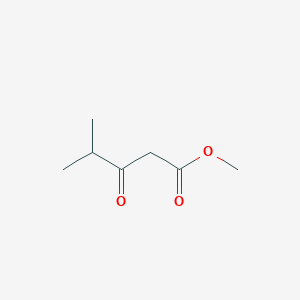
Methyl 4-methyl-3-oxopentanoate
Overview
Description
Methyl 4-methyl-3-oxopentanoate is an organic compound with the molecular formula C7H12O3. It is a methyl ester derivative of 4-methyl-3-oxopentanoic acid and is commonly used in various research fields due to its unique chemical properties .
Preparation Methods
Synthetic Routes and Reaction Conditions
Methyl 4-methyl-3-oxopentanoate can be synthesized through several methods. One common method involves the esterification of 4-methyl-3-oxopentanoic acid with methanol in the presence of an acid catalyst such as sulfuric acid. The reaction is typically carried out under reflux conditions to ensure complete conversion .
Industrial Production Methods
In industrial settings, the production of this compound often involves the use of continuous flow reactors to optimize yield and efficiency. The process may include steps such as distillation and purification to obtain a high-purity product .
Chemical Reactions Analysis
Types of Reactions
Methyl 4-methyl-3-oxopentanoate undergoes various chemical reactions, including:
Oxidation: It can be oxidized to form corresponding carboxylic acids.
Reduction: Reduction reactions can convert it into alcohols.
Substitution: It can undergo nucleophilic substitution reactions, where the ester group is replaced by other functional groups.
Common Reagents and Conditions
Oxidation: Common oxidizing agents include potassium permanganate (KMnO4) and chromium trioxide (CrO3).
Reduction: Reducing agents such as lithium aluminum hydride (LiAlH4) and sodium borohydride (NaBH4) are often used.
Substitution: Nucleophiles like amines and alcohols can be used under basic or acidic conditions.
Major Products Formed
Oxidation: 4-methyl-3-oxopentanoic acid.
Reduction: 4-methyl-3-hydroxypentanoate.
Substitution: Various substituted esters depending on the nucleophile used.
Scientific Research Applications
Methyl 4-methyl-3-oxopentanoate is widely used in scientific research due to its versatility:
Chemistry: It serves as a building block in organic synthesis and is used in the preparation of various complex molecules.
Biology: It is used in studies related to enzyme activity and metabolic pathways.
Medicine: Research involving drug development and pharmacokinetics often utilizes this compound.
Industry: It is used in the production of fragrances, flavors, and other fine chemicals
Mechanism of Action
The mechanism of action of methyl 4-methyl-3-oxopentanoate involves its interaction with various molecular targets. In biological systems, it can act as a substrate for enzymes, leading to the formation of different metabolites. The pathways involved often include ester hydrolysis and subsequent oxidation or reduction reactions .
Comparison with Similar Compounds
Similar Compounds
Methyl 3-methyl-4-oxopentanoate: Similar in structure but differs in the position of the methyl group.
Methyl 4,4-dimethyl-3-oxopentanoate: Contains an additional methyl group, leading to different chemical properties
Uniqueness
Methyl 4-methyl-3-oxopentanoate is unique due to its specific structural configuration, which imparts distinct reactivity and stability compared to its analogs. This makes it particularly useful in targeted synthetic applications and research studies .
Properties
IUPAC Name |
methyl 4-methyl-3-oxopentanoate | |
---|---|---|
Source | PubChem | |
URL | https://pubchem.ncbi.nlm.nih.gov | |
Description | Data deposited in or computed by PubChem | |
InChI |
InChI=1S/C7H12O3/c1-5(2)6(8)4-7(9)10-3/h5H,4H2,1-3H3 | |
Source | PubChem | |
URL | https://pubchem.ncbi.nlm.nih.gov | |
Description | Data deposited in or computed by PubChem | |
InChI Key |
HNNFDXWDCFCVDM-UHFFFAOYSA-N | |
Source | PubChem | |
URL | https://pubchem.ncbi.nlm.nih.gov | |
Description | Data deposited in or computed by PubChem | |
Canonical SMILES |
CC(C)C(=O)CC(=O)OC | |
Source | PubChem | |
URL | https://pubchem.ncbi.nlm.nih.gov | |
Description | Data deposited in or computed by PubChem | |
Molecular Formula |
C7H12O3 | |
Source | PubChem | |
URL | https://pubchem.ncbi.nlm.nih.gov | |
Description | Data deposited in or computed by PubChem | |
DSSTOX Substance ID |
DTXSID40374974 | |
Record name | Methyl isobutyrylacetate | |
Source | EPA DSSTox | |
URL | https://comptox.epa.gov/dashboard/DTXSID40374974 | |
Description | DSSTox provides a high quality public chemistry resource for supporting improved predictive toxicology. | |
Molecular Weight |
144.17 g/mol | |
Source | PubChem | |
URL | https://pubchem.ncbi.nlm.nih.gov | |
Description | Data deposited in or computed by PubChem | |
CAS No. |
42558-54-3 | |
Record name | Pentanoic acid, 4-methyl-3-oxo-, methyl ester | |
Source | CAS Common Chemistry | |
URL | https://commonchemistry.cas.org/detail?cas_rn=42558-54-3 | |
Description | CAS Common Chemistry is an open community resource for accessing chemical information. Nearly 500,000 chemical substances from CAS REGISTRY cover areas of community interest, including common and frequently regulated chemicals, and those relevant to high school and undergraduate chemistry classes. This chemical information, curated by our expert scientists, is provided in alignment with our mission as a division of the American Chemical Society. | |
Explanation | The data from CAS Common Chemistry is provided under a CC-BY-NC 4.0 license, unless otherwise stated. | |
Record name | Methyl 4-methyl-3-oxopentanoate | |
Source | ChemIDplus | |
URL | https://pubchem.ncbi.nlm.nih.gov/substance/?source=chemidplus&sourceid=0042558543 | |
Description | ChemIDplus is a free, web search system that provides access to the structure and nomenclature authority files used for the identification of chemical substances cited in National Library of Medicine (NLM) databases, including the TOXNET system. | |
Record name | Methyl isobutyrylacetate | |
Source | EPA DSSTox | |
URL | https://comptox.epa.gov/dashboard/DTXSID40374974 | |
Description | DSSTox provides a high quality public chemistry resource for supporting improved predictive toxicology. | |
Record name | Methyl 4-methyl-3-oxopentanoate | |
Source | European Chemicals Agency (ECHA) | |
URL | https://echa.europa.eu/substance-information/-/substanceinfo/100.101.768 | |
Description | The European Chemicals Agency (ECHA) is an agency of the European Union which is the driving force among regulatory authorities in implementing the EU's groundbreaking chemicals legislation for the benefit of human health and the environment as well as for innovation and competitiveness. | |
Explanation | Use of the information, documents and data from the ECHA website is subject to the terms and conditions of this Legal Notice, and subject to other binding limitations provided for under applicable law, the information, documents and data made available on the ECHA website may be reproduced, distributed and/or used, totally or in part, for non-commercial purposes provided that ECHA is acknowledged as the source: "Source: European Chemicals Agency, http://echa.europa.eu/". Such acknowledgement must be included in each copy of the material. ECHA permits and encourages organisations and individuals to create links to the ECHA website under the following cumulative conditions: Links can only be made to webpages that provide a link to the Legal Notice page. | |
Synthesis routes and methods
Procedure details
Retrosynthesis Analysis
AI-Powered Synthesis Planning: Our tool employs the Template_relevance Pistachio, Template_relevance Bkms_metabolic, Template_relevance Pistachio_ringbreaker, Template_relevance Reaxys, Template_relevance Reaxys_biocatalysis model, leveraging a vast database of chemical reactions to predict feasible synthetic routes.
One-Step Synthesis Focus: Specifically designed for one-step synthesis, it provides concise and direct routes for your target compounds, streamlining the synthesis process.
Accurate Predictions: Utilizing the extensive PISTACHIO, BKMS_METABOLIC, PISTACHIO_RINGBREAKER, REAXYS, REAXYS_BIOCATALYSIS database, our tool offers high-accuracy predictions, reflecting the latest in chemical research and data.
Strategy Settings
Precursor scoring | Relevance Heuristic |
---|---|
Min. plausibility | 0.01 |
Model | Template_relevance |
Template Set | Pistachio/Bkms_metabolic/Pistachio_ringbreaker/Reaxys/Reaxys_biocatalysis |
Top-N result to add to graph | 6 |
Feasible Synthetic Routes
Q1: What is the role of Methyl 4-methyl-3-oxopentanoate in the synthesis of new compounds?
A1: this compound serves as a valuable starting material in the synthesis of various chemical compounds. For example, it is used in the preparation of pyrazoline analogues of Rosuvastatin []. These analogues are being investigated for their potential antimicrobial and anti-inflammatory properties. The structure of this compound allows for modifications at different positions, making it a versatile building block for creating diverse chemical structures.
Q2: How is this compound used in enzymatic reactions?
A2: this compound acts as a substrate in enzymatic transesterification reactions catalyzed by immobilized Candida antarctica lipase B []. This reaction is being studied for the synthesis of Butyl-4-methyl-3-oxopentanoate, a compound with potential applications in various fields. Researchers are exploring the optimization of this reaction using response surface methodology to enhance the efficiency and yield of Butyl-4-methyl-3-oxopentanoate production.
Disclaimer and Information on In-Vitro Research Products
Please be aware that all articles and product information presented on BenchChem are intended solely for informational purposes. The products available for purchase on BenchChem are specifically designed for in-vitro studies, which are conducted outside of living organisms. In-vitro studies, derived from the Latin term "in glass," involve experiments performed in controlled laboratory settings using cells or tissues. It is important to note that these products are not categorized as medicines or drugs, and they have not received approval from the FDA for the prevention, treatment, or cure of any medical condition, ailment, or disease. We must emphasize that any form of bodily introduction of these products into humans or animals is strictly prohibited by law. It is essential to adhere to these guidelines to ensure compliance with legal and ethical standards in research and experimentation.