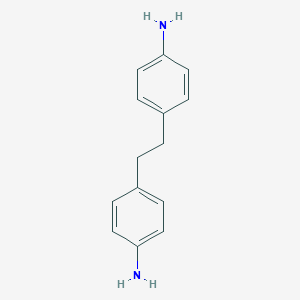
4,4'-Ethylenedianiline
Overview
Description
It is a white to gray to brown crystalline solid that is soluble in some organic solvents such as ethanol, anhydrous ether, and dimethylformamide . This compound is primarily used as an intermediate in the synthesis of dyes, pigments, and other organic compounds.
Mechanism of Action
Target of Action
It’s known that this compound is often used in the synthesis of various organic compounds, suggesting that its targets could be a wide range of organic molecules .
Mode of Action
It may interact with its targets through covalent bonding, leading to the formation of new compounds .
Biochemical Pathways
It’s used in the synthesis of various organic compounds, suggesting that it could potentially affect a wide range of biochemical pathways depending on the specific compounds it’s used to synthesize .
Pharmacokinetics
As such, its impact on bioavailability is currently unknown .
Result of Action
Given its use in the synthesis of various organic compounds, it’s likely that its effects would vary depending on the specific compounds it’s used to synthesize .
Action Environment
The action, efficacy, and stability of 4,4’-Ethylenedianiline can be influenced by various environmental factors. For instance, its reactivity may be affected by factors such as temperature, pH, and the presence of other chemicals. Proper storage conditions are necessary to maintain its stability .
Biochemical Analysis
Biochemical Properties
It is known that the compound can interact with various enzymes, proteins, and other biomolecules in the process of polyurethane production
Molecular Mechanism
It is known that the compound is involved in the production of polyurethane, a process that likely involves binding interactions with biomolecules, potential enzyme inhibition or activation, and changes in gene expression
Metabolic Pathways
It is known that the compound is used in the production of polyurethane
Preparation Methods
Synthetic Routes and Reaction Conditions
One common method for synthesizing 4,4’-Ethylenedianiline involves the reduction of 4,4’-dinitrobibenzyl using hydrogen gas in the presence of a palladium catalyst on activated carbon. The reaction is typically carried out in methanol at room temperature, and the product is obtained in high yield after filtration and recrystallization .
Industrial Production Methods
In industrial settings, 4,4’-Ethylenedianiline is often produced by the catalytic hydrogenation of 4,4’-dinitrobibenzyl. This process involves the use of a continuous flow reactor where hydrogen gas and the nitro compound are passed over a palladium catalyst supported on activated carbon. The reaction is conducted under controlled temperature and pressure conditions to ensure high efficiency and yield .
Chemical Reactions Analysis
Types of Reactions
4,4’-Ethylenedianiline undergoes various chemical reactions, including:
Oxidation: It can be oxidized to form corresponding quinones.
Reduction: The compound can be reduced to form 4,4’-diaminodicyclohexylmethane.
Substitution: It can participate in electrophilic aromatic substitution reactions, such as nitration and sulfonation.
Common Reagents and Conditions
Oxidation: Common oxidizing agents include potassium permanganate and chromium trioxide.
Reduction: Hydrogen gas in the presence of a palladium catalyst is commonly used.
Substitution: Reagents such as nitric acid for nitration and sulfuric acid for sulfonation are typically employed.
Major Products Formed
Oxidation: Quinones.
Reduction: 4,4’-Diaminodicyclohexylmethane.
Substitution: Nitro and sulfonic acid derivatives.
Scientific Research Applications
Comparison with Similar Compounds
Similar Compounds
4,4’-Methylenedianiline: Similar in structure but has a methylene bridge instead of an ethylene bridge.
4,4’-Diaminodiphenylmethane: Another similar compound with a methylene bridge.
4,4’-Oxydianiline: Contains an oxygen atom linking the two aromatic rings instead of an ethylene bridge.
Uniqueness
4,4’-Ethylenedianiline is unique due to its ethylene bridge, which imparts distinct chemical and physical properties compared to its analogs. This structural difference influences its reactivity and the types of interactions it can participate in, making it a valuable compound in various chemical and industrial applications .
Properties
IUPAC Name |
4-[2-(4-aminophenyl)ethyl]aniline | |
---|---|---|
Source | PubChem | |
URL | https://pubchem.ncbi.nlm.nih.gov | |
Description | Data deposited in or computed by PubChem | |
InChI |
InChI=1S/C14H16N2/c15-13-7-3-11(4-8-13)1-2-12-5-9-14(16)10-6-12/h3-10H,1-2,15-16H2 | |
Source | PubChem | |
URL | https://pubchem.ncbi.nlm.nih.gov | |
Description | Data deposited in or computed by PubChem | |
InChI Key |
UHNUHZHQLCGZDA-UHFFFAOYSA-N | |
Source | PubChem | |
URL | https://pubchem.ncbi.nlm.nih.gov | |
Description | Data deposited in or computed by PubChem | |
Canonical SMILES |
C1=CC(=CC=C1CCC2=CC=C(C=C2)N)N | |
Source | PubChem | |
URL | https://pubchem.ncbi.nlm.nih.gov | |
Description | Data deposited in or computed by PubChem | |
Molecular Formula |
C14H16N2 | |
Source | PubChem | |
URL | https://pubchem.ncbi.nlm.nih.gov | |
Description | Data deposited in or computed by PubChem | |
DSSTOX Substance ID |
DTXSID3060742 | |
Record name | Benzenamine, 4,4'-(1,2-ethanediyl)bis- | |
Source | EPA DSSTox | |
URL | https://comptox.epa.gov/dashboard/DTXSID3060742 | |
Description | DSSTox provides a high quality public chemistry resource for supporting improved predictive toxicology. | |
Molecular Weight |
212.29 g/mol | |
Source | PubChem | |
URL | https://pubchem.ncbi.nlm.nih.gov | |
Description | Data deposited in or computed by PubChem | |
Solubility |
22 [ug/mL] (The mean of the results at pH 7.4) | |
Record name | SID47195535 | |
Source | Burnham Center for Chemical Genomics | |
URL | https://pubchem.ncbi.nlm.nih.gov/bioassay/1996#section=Data-Table | |
Description | Aqueous solubility in buffer at pH 7.4 | |
CAS No. |
621-95-4 | |
Record name | 4,4′-Ethylenedianiline | |
Source | CAS Common Chemistry | |
URL | https://commonchemistry.cas.org/detail?cas_rn=621-95-4 | |
Description | CAS Common Chemistry is an open community resource for accessing chemical information. Nearly 500,000 chemical substances from CAS REGISTRY cover areas of community interest, including common and frequently regulated chemicals, and those relevant to high school and undergraduate chemistry classes. This chemical information, curated by our expert scientists, is provided in alignment with our mission as a division of the American Chemical Society. | |
Explanation | The data from CAS Common Chemistry is provided under a CC-BY-NC 4.0 license, unless otherwise stated. | |
Record name | 4,4'-Diaminodibenzyl | |
Source | ChemIDplus | |
URL | https://pubchem.ncbi.nlm.nih.gov/substance/?source=chemidplus&sourceid=0000621954 | |
Description | ChemIDplus is a free, web search system that provides access to the structure and nomenclature authority files used for the identification of chemical substances cited in National Library of Medicine (NLM) databases, including the TOXNET system. | |
Record name | 4,4'-Diaminobibenzyl | |
Source | DTP/NCI | |
URL | https://dtp.cancer.gov/dtpstandard/servlet/dwindex?searchtype=NSC&outputformat=html&searchlist=60033 | |
Description | The NCI Development Therapeutics Program (DTP) provides services and resources to the academic and private-sector research communities worldwide to facilitate the discovery and development of new cancer therapeutic agents. | |
Explanation | Unless otherwise indicated, all text within NCI products is free of copyright and may be reused without our permission. Credit the National Cancer Institute as the source. | |
Record name | 4,4'-Diaminobibenzyl | |
Source | DTP/NCI | |
URL | https://dtp.cancer.gov/dtpstandard/servlet/dwindex?searchtype=NSC&outputformat=html&searchlist=44062 | |
Description | The NCI Development Therapeutics Program (DTP) provides services and resources to the academic and private-sector research communities worldwide to facilitate the discovery and development of new cancer therapeutic agents. | |
Explanation | Unless otherwise indicated, all text within NCI products is free of copyright and may be reused without our permission. Credit the National Cancer Institute as the source. | |
Record name | 4,4'-Diaminobibenzyl | |
Source | DTP/NCI | |
URL | https://dtp.cancer.gov/dtpstandard/servlet/dwindex?searchtype=NSC&outputformat=html&searchlist=580 | |
Description | The NCI Development Therapeutics Program (DTP) provides services and resources to the academic and private-sector research communities worldwide to facilitate the discovery and development of new cancer therapeutic agents. | |
Explanation | Unless otherwise indicated, all text within NCI products is free of copyright and may be reused without our permission. Credit the National Cancer Institute as the source. | |
Record name | Benzenamine, 4,4'-(1,2-ethanediyl)bis- | |
Source | EPA Chemicals under the TSCA | |
URL | https://www.epa.gov/chemicals-under-tsca | |
Description | EPA Chemicals under the Toxic Substances Control Act (TSCA) collection contains information on chemicals and their regulations under TSCA, including non-confidential content from the TSCA Chemical Substance Inventory and Chemical Data Reporting. | |
Record name | Benzenamine, 4,4'-(1,2-ethanediyl)bis- | |
Source | EPA DSSTox | |
URL | https://comptox.epa.gov/dashboard/DTXSID3060742 | |
Description | DSSTox provides a high quality public chemistry resource for supporting improved predictive toxicology. | |
Record name | 4,4'-ethylenedianiline | |
Source | European Chemicals Agency (ECHA) | |
URL | https://echa.europa.eu/substance-information/-/substanceinfo/100.009.743 | |
Description | The European Chemicals Agency (ECHA) is an agency of the European Union which is the driving force among regulatory authorities in implementing the EU's groundbreaking chemicals legislation for the benefit of human health and the environment as well as for innovation and competitiveness. | |
Explanation | Use of the information, documents and data from the ECHA website is subject to the terms and conditions of this Legal Notice, and subject to other binding limitations provided for under applicable law, the information, documents and data made available on the ECHA website may be reproduced, distributed and/or used, totally or in part, for non-commercial purposes provided that ECHA is acknowledged as the source: "Source: European Chemicals Agency, http://echa.europa.eu/". Such acknowledgement must be included in each copy of the material. ECHA permits and encourages organisations and individuals to create links to the ECHA website under the following cumulative conditions: Links can only be made to webpages that provide a link to the Legal Notice page. | |
Retrosynthesis Analysis
AI-Powered Synthesis Planning: Our tool employs the Template_relevance Pistachio, Template_relevance Bkms_metabolic, Template_relevance Pistachio_ringbreaker, Template_relevance Reaxys, Template_relevance Reaxys_biocatalysis model, leveraging a vast database of chemical reactions to predict feasible synthetic routes.
One-Step Synthesis Focus: Specifically designed for one-step synthesis, it provides concise and direct routes for your target compounds, streamlining the synthesis process.
Accurate Predictions: Utilizing the extensive PISTACHIO, BKMS_METABOLIC, PISTACHIO_RINGBREAKER, REAXYS, REAXYS_BIOCATALYSIS database, our tool offers high-accuracy predictions, reflecting the latest in chemical research and data.
Strategy Settings
Precursor scoring | Relevance Heuristic |
---|---|
Min. plausibility | 0.01 |
Model | Template_relevance |
Template Set | Pistachio/Bkms_metabolic/Pistachio_ringbreaker/Reaxys/Reaxys_biocatalysis |
Top-N result to add to graph | 6 |
Feasible Synthetic Routes
Disclaimer and Information on In-Vitro Research Products
Please be aware that all articles and product information presented on BenchChem are intended solely for informational purposes. The products available for purchase on BenchChem are specifically designed for in-vitro studies, which are conducted outside of living organisms. In-vitro studies, derived from the Latin term "in glass," involve experiments performed in controlled laboratory settings using cells or tissues. It is important to note that these products are not categorized as medicines or drugs, and they have not received approval from the FDA for the prevention, treatment, or cure of any medical condition, ailment, or disease. We must emphasize that any form of bodily introduction of these products into humans or animals is strictly prohibited by law. It is essential to adhere to these guidelines to ensure compliance with legal and ethical standards in research and experimentation.