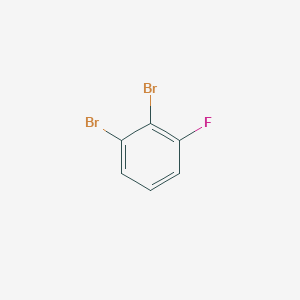
1,2-Dibromo-3-fluorobenzene
Overview
Description
1,2-Dibromo-3-fluorobenzene is an organic compound with the molecular formula C₆H₃Br₂F. It is a derivative of benzene, where two bromine atoms and one fluorine atom are substituted at the 1, 2, and 3 positions, respectively. This compound is a colorless liquid or solid, depending on the temperature, and is used in various chemical synthesis processes .
Biochemical Analysis
Biochemical Properties
It is known that the bromine atoms in the compound serve as reactive sites for further functionalization through various cross-coupling reactions. These reactions are pivotal in creating biaryl structures often found in pharmaceuticals.
Cellular Effects
It is known that the compound can cause skin irritation, serious eye irritation, and may cause respiratory irritation .
Molecular Mechanism
Temporal Effects in Laboratory Settings
Metabolic Pathways
Preparation Methods
Synthetic Routes and Reaction Conditions
1,2-Dibromo-3-fluorobenzene can be synthesized through several methods. One common method involves the bromination of 3-fluorobenzene using bromine in the presence of a catalyst such as iron or aluminum bromide. The reaction typically occurs at room temperature and yields this compound as the primary product .
Industrial Production Methods
In industrial settings, the production of this compound may involve continuous flow processes to ensure higher yields and purity. The use of tubular diazotization reaction technology is one such method, which offers advantages such as reduced side reactions, improved stability, and higher yields .
Chemical Reactions Analysis
Types of Reactions
1,2-Dibromo-3-fluorobenzene undergoes various chemical reactions, including:
Substitution Reactions: It can participate in nucleophilic aromatic substitution reactions where the bromine atoms are replaced by other nucleophiles.
Coupling Reactions: It is commonly used in Suzuki-Miyaura coupling reactions to form carbon-carbon bonds.
Common Reagents and Conditions
Nucleophilic Aromatic Substitution: Reagents such as sodium methoxide or potassium tert-butoxide can be used under basic conditions.
Suzuki-Miyaura Coupling: Palladium catalysts and boron reagents are typically used under mild conditions.
Major Products Formed
Substitution Reactions: Products depend on the nucleophile used, such as fluorinated or brominated derivatives.
Coupling Reactions: Formation of biaryl compounds or other complex aromatic structures.
Scientific Research Applications
1,2-Dibromo-3-fluorobenzene has several applications in scientific research:
Chemistry: It is used as a building block in the synthesis of more complex organic molecules.
Biology: It can be used in the study of enzyme interactions and inhibition.
Industry: Used in the production of specialty chemicals and materials
Mechanism of Action
The mechanism of action of 1,2-dibromo-3-fluorobenzene in chemical reactions involves electrophilic aromatic substitution. The bromine atoms act as leaving groups, allowing nucleophiles to attack the aromatic ring. This process typically involves the formation of a positively charged benzenonium intermediate, followed by the removal of a proton to restore aromaticity .
Comparison with Similar Compounds
Similar Compounds
- 1,3-Dibromo-2-fluorobenzene
- 1,4-Dibromo-2-fluorobenzene
- 1,2-Dibromo-4-fluorobenzene
Uniqueness
1,2-Dibromo-3-fluorobenzene is unique due to the specific positioning of the bromine and fluorine atoms on the benzene ring. This positioning influences its reactivity and the types of reactions it can undergo compared to other isomers .
Properties
IUPAC Name |
1,2-dibromo-3-fluorobenzene | |
---|---|---|
Source | PubChem | |
URL | https://pubchem.ncbi.nlm.nih.gov | |
Description | Data deposited in or computed by PubChem | |
InChI |
InChI=1S/C6H3Br2F/c7-4-2-1-3-5(9)6(4)8/h1-3H | |
Source | PubChem | |
URL | https://pubchem.ncbi.nlm.nih.gov | |
Description | Data deposited in or computed by PubChem | |
InChI Key |
DWNYXIICDFVJEX-UHFFFAOYSA-N | |
Source | PubChem | |
URL | https://pubchem.ncbi.nlm.nih.gov | |
Description | Data deposited in or computed by PubChem | |
Canonical SMILES |
C1=CC(=C(C(=C1)Br)Br)F | |
Source | PubChem | |
URL | https://pubchem.ncbi.nlm.nih.gov | |
Description | Data deposited in or computed by PubChem | |
Molecular Formula |
C6H3Br2F | |
Source | PubChem | |
URL | https://pubchem.ncbi.nlm.nih.gov | |
Description | Data deposited in or computed by PubChem | |
DSSTOX Substance ID |
DTXSID401001821 | |
Record name | 1,2-Dibromo-3-fluorobenzene | |
Source | EPA DSSTox | |
URL | https://comptox.epa.gov/dashboard/DTXSID401001821 | |
Description | DSSTox provides a high quality public chemistry resource for supporting improved predictive toxicology. | |
Molecular Weight |
253.89 g/mol | |
Source | PubChem | |
URL | https://pubchem.ncbi.nlm.nih.gov | |
Description | Data deposited in or computed by PubChem | |
CAS No. |
811711-33-8 | |
Record name | 1,2-Dibromo-3-fluorobenzene | |
Source | EPA DSSTox | |
URL | https://comptox.epa.gov/dashboard/DTXSID401001821 | |
Description | DSSTox provides a high quality public chemistry resource for supporting improved predictive toxicology. | |
Retrosynthesis Analysis
AI-Powered Synthesis Planning: Our tool employs the Template_relevance Pistachio, Template_relevance Bkms_metabolic, Template_relevance Pistachio_ringbreaker, Template_relevance Reaxys, Template_relevance Reaxys_biocatalysis model, leveraging a vast database of chemical reactions to predict feasible synthetic routes.
One-Step Synthesis Focus: Specifically designed for one-step synthesis, it provides concise and direct routes for your target compounds, streamlining the synthesis process.
Accurate Predictions: Utilizing the extensive PISTACHIO, BKMS_METABOLIC, PISTACHIO_RINGBREAKER, REAXYS, REAXYS_BIOCATALYSIS database, our tool offers high-accuracy predictions, reflecting the latest in chemical research and data.
Strategy Settings
Precursor scoring | Relevance Heuristic |
---|---|
Min. plausibility | 0.01 |
Model | Template_relevance |
Template Set | Pistachio/Bkms_metabolic/Pistachio_ringbreaker/Reaxys/Reaxys_biocatalysis |
Top-N result to add to graph | 6 |
Feasible Synthetic Routes
Disclaimer and Information on In-Vitro Research Products
Please be aware that all articles and product information presented on BenchChem are intended solely for informational purposes. The products available for purchase on BenchChem are specifically designed for in-vitro studies, which are conducted outside of living organisms. In-vitro studies, derived from the Latin term "in glass," involve experiments performed in controlled laboratory settings using cells or tissues. It is important to note that these products are not categorized as medicines or drugs, and they have not received approval from the FDA for the prevention, treatment, or cure of any medical condition, ailment, or disease. We must emphasize that any form of bodily introduction of these products into humans or animals is strictly prohibited by law. It is essential to adhere to these guidelines to ensure compliance with legal and ethical standards in research and experimentation.