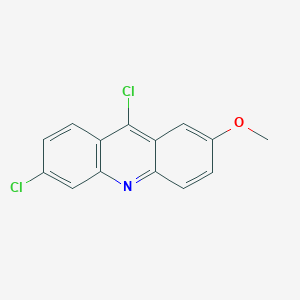
6,9-Dichloro-2-methoxyacridine
Overview
Description
6,9-Dichloro-2-methoxyacridine is used to prepare 6-chloro-2-methoxy-acridin-9-ylamine by reacting with phenol and ammonium carbonate . It is also used in the preparation of 9-amino-6-chloro-2-methoxyacridine, N’- (6-Chloro-2-methoxy-acridin-9-yl)-heptylamine and N,N’-bis- (6-chloro-2-methoxy-acridin-9-yl)-hexane-1,6-diamine .
Synthesis Analysis
6,9-Dichloro-2-methoxyacridine is synthesized by reacting with phenol and ammonium carbonate . It is used in the preparation of 9-amino-6-chloro-2-methoxyacridine, N’- (6-Chloro-2-methoxy-acridin-9-yl)-heptylamine and N,N’-bis- (6-chloro-2-methoxy-acridin-9-yl)-hexane-1,6-diamine .Molecular Structure Analysis
The structure of 6,9-dichloro-2-methoxyacridine consists of centrosymmetrically related, stacked molecules . The acridine nucleus is slightly buckled, and does not show mirror symmetry along the C (9)-N (10) line .Chemical Reactions Analysis
6,9-Dichloro-2-methoxyacridine on reaction with quinolizidinylalkylamines yields 4-aminoquinoline and 9-aminoacridine derivatives .Physical And Chemical Properties Analysis
The molecular weight of 6,9-Dichloro-2-methoxyacridine is 278.1 g/mol . It has a melting point of 163-165 °C (lit.) . The compound is insoluble in water .Scientific Research Applications
Comprehensive Analysis of 6,9-Dichloro-2-methoxyacridine Applications
6,9-Dichloro-2-methoxyacridine (C14H9Cl2NO) is a compound with several applications in scientific research. Below is a detailed analysis of its unique applications across different fields.
Synthesis of Aminoacridines: 6,9-Dichloro-2-methoxyacridine is used as a precursor in the synthesis of 9-amino-6-chloro-2-methoxyacridine . This compound is significant in the development of new pharmaceuticals, particularly in the creation of drugs with potential antimalarial properties .
Preparation of Acridinylamines: The compound is involved in the preparation of 6-chloro-2-methoxy-acridin-9-ylamine . This reaction typically involves phenol and ammonium carbonate, leading to intermediates useful in further chemical synthesis .
Development of Heptylamine Derivatives: 6,9-Dichloro-2-methoxyacridine reacts to form N′-(6-Chloro-2-methoxy-acridin-9-yl)-heptylamine . These derivatives are studied for their potential use in biochemical applications, including as ligands for biological receptors .
Creation of Hexane-1,6-diamine Derivatives: Another application is the formation of N,N′-bis-(6-chloro-2-methoxy-acridin-9-yl)-hexane-1,6-diamine . Such compounds are of interest in materials science for their unique structural and electronic properties .
Production of 4-Aminoquinoline Derivatives: By reacting with quinolizidinylalkylamines, 6,9-Dichloro-2-methoxyacridine is used to produce 4-aminoquinoline derivatives . These derivatives are important in medicinal chemistry, particularly in the synthesis of antimalarial drugs .
Generation of 9-Aminoacridine Derivatives: The compound is also instrumental in generating 9-aminoacridine derivatives . These derivatives have applications in the development of antiseptic agents and in the study of DNA intercalation .
Research in Antimalarial Activity: Due to its role in synthesizing various acridine derivatives, 6,9-Dichloro-2-methoxyacridine is central to research focused on antimalarial activity. Its derivatives are being explored for their efficacy against malaria parasites .
Exploration in Biochemical Sensing: Finally, the compound’s derivatives are being explored for their use in biochemical sensing mechanisms. This includes potential applications in detecting biological markers and in the development of diagnostic tools .
Safety and Hazards
Mechanism of Action
Target of Action
It’s known that the compound is used in the synthesis of 4-aminoquinoline and 9-aminoacridine derivatives , which are of interest as potential antibacterial, antiprotozoarian, anti-helminthic, and antitumoral agents .
Mode of Action
It’s known that the compound reacts with quinolizidinylalkylamines to yield 4-aminoquinoline and 9-aminoacridine derivatives . These derivatives are believed to interact with their targets, leading to their potential antibacterial, antiprotozoarian, anti-helminthic, and antitumoral effects .
Biochemical Pathways
Given its role in the synthesis of 4-aminoquinoline and 9-aminoacridine derivatives , it’s plausible that the compound may influence pathways related to these derivatives.
properties
IUPAC Name |
6,9-dichloro-2-methoxyacridine | |
---|---|---|
Source | PubChem | |
URL | https://pubchem.ncbi.nlm.nih.gov | |
Description | Data deposited in or computed by PubChem | |
InChI |
InChI=1S/C14H9Cl2NO/c1-18-9-3-5-12-11(7-9)14(16)10-4-2-8(15)6-13(10)17-12/h2-7H,1H3 | |
Source | PubChem | |
URL | https://pubchem.ncbi.nlm.nih.gov | |
Description | Data deposited in or computed by PubChem | |
InChI Key |
RYRNQWYNHLLOGX-UHFFFAOYSA-N | |
Source | PubChem | |
URL | https://pubchem.ncbi.nlm.nih.gov | |
Description | Data deposited in or computed by PubChem | |
Canonical SMILES |
COC1=CC2=C(C3=C(C=C(C=C3)Cl)N=C2C=C1)Cl | |
Source | PubChem | |
URL | https://pubchem.ncbi.nlm.nih.gov | |
Description | Data deposited in or computed by PubChem | |
Molecular Formula |
C14H9Cl2NO | |
Source | PubChem | |
URL | https://pubchem.ncbi.nlm.nih.gov | |
Description | Data deposited in or computed by PubChem | |
DSSTOX Substance ID |
DTXSID60235331 | |
Record name | 3,9-Dichloro-7-methoxyacridine | |
Source | EPA DSSTox | |
URL | https://comptox.epa.gov/dashboard/DTXSID60235331 | |
Description | DSSTox provides a high quality public chemistry resource for supporting improved predictive toxicology. | |
Molecular Weight |
278.1 g/mol | |
Source | PubChem | |
URL | https://pubchem.ncbi.nlm.nih.gov | |
Description | Data deposited in or computed by PubChem | |
Product Name |
6,9-Dichloro-2-methoxyacridine | |
CAS RN |
86-38-4 | |
Record name | 6,9-Dichloro-2-methoxyacridine | |
Source | CAS Common Chemistry | |
URL | https://commonchemistry.cas.org/detail?cas_rn=86-38-4 | |
Description | CAS Common Chemistry is an open community resource for accessing chemical information. Nearly 500,000 chemical substances from CAS REGISTRY cover areas of community interest, including common and frequently regulated chemicals, and those relevant to high school and undergraduate chemistry classes. This chemical information, curated by our expert scientists, is provided in alignment with our mission as a division of the American Chemical Society. | |
Explanation | The data from CAS Common Chemistry is provided under a CC-BY-NC 4.0 license, unless otherwise stated. | |
Record name | 3,9-Dichloro-7-methoxyacridine | |
Source | ChemIDplus | |
URL | https://pubchem.ncbi.nlm.nih.gov/substance/?source=chemidplus&sourceid=0000086384 | |
Description | ChemIDplus is a free, web search system that provides access to the structure and nomenclature authority files used for the identification of chemical substances cited in National Library of Medicine (NLM) databases, including the TOXNET system. | |
Record name | 6,9-Dichloro-2-methoxyacridine | |
Source | DTP/NCI | |
URL | https://dtp.cancer.gov/dtpstandard/servlet/dwindex?searchtype=NSC&outputformat=html&searchlist=2951 | |
Description | The NCI Development Therapeutics Program (DTP) provides services and resources to the academic and private-sector research communities worldwide to facilitate the discovery and development of new cancer therapeutic agents. | |
Explanation | Unless otherwise indicated, all text within NCI products is free of copyright and may be reused without our permission. Credit the National Cancer Institute as the source. | |
Record name | 6,9-Dichloro-2-methoxyacridine | |
Source | DTP/NCI | |
URL | https://dtp.cancer.gov/dtpstandard/servlet/dwindex?searchtype=NSC&outputformat=html&searchlist=2095 | |
Description | The NCI Development Therapeutics Program (DTP) provides services and resources to the academic and private-sector research communities worldwide to facilitate the discovery and development of new cancer therapeutic agents. | |
Explanation | Unless otherwise indicated, all text within NCI products is free of copyright and may be reused without our permission. Credit the National Cancer Institute as the source. | |
Record name | 3,9-Dichloro-7-methoxyacridine | |
Source | EPA DSSTox | |
URL | https://comptox.epa.gov/dashboard/DTXSID60235331 | |
Description | DSSTox provides a high quality public chemistry resource for supporting improved predictive toxicology. | |
Record name | 3,9-dichloro-7-methoxyacridine | |
Source | European Chemicals Agency (ECHA) | |
URL | https://echa.europa.eu/substance-information/-/substanceinfo/100.001.515 | |
Description | The European Chemicals Agency (ECHA) is an agency of the European Union which is the driving force among regulatory authorities in implementing the EU's groundbreaking chemicals legislation for the benefit of human health and the environment as well as for innovation and competitiveness. | |
Explanation | Use of the information, documents and data from the ECHA website is subject to the terms and conditions of this Legal Notice, and subject to other binding limitations provided for under applicable law, the information, documents and data made available on the ECHA website may be reproduced, distributed and/or used, totally or in part, for non-commercial purposes provided that ECHA is acknowledged as the source: "Source: European Chemicals Agency, http://echa.europa.eu/". Such acknowledgement must be included in each copy of the material. ECHA permits and encourages organisations and individuals to create links to the ECHA website under the following cumulative conditions: Links can only be made to webpages that provide a link to the Legal Notice page. | |
Record name | 3,9-DICHLORO-7-METHOXYACRIDINE | |
Source | FDA Global Substance Registration System (GSRS) | |
URL | https://gsrs.ncats.nih.gov/ginas/app/beta/substances/L7V65XW6XU | |
Description | The FDA Global Substance Registration System (GSRS) enables the efficient and accurate exchange of information on what substances are in regulated products. Instead of relying on names, which vary across regulatory domains, countries, and regions, the GSRS knowledge base makes it possible for substances to be defined by standardized, scientific descriptions. | |
Explanation | Unless otherwise noted, the contents of the FDA website (www.fda.gov), both text and graphics, are not copyrighted. They are in the public domain and may be republished, reprinted and otherwise used freely by anyone without the need to obtain permission from FDA. Credit to the U.S. Food and Drug Administration as the source is appreciated but not required. | |
Retrosynthesis Analysis
AI-Powered Synthesis Planning: Our tool employs the Template_relevance Pistachio, Template_relevance Bkms_metabolic, Template_relevance Pistachio_ringbreaker, Template_relevance Reaxys, Template_relevance Reaxys_biocatalysis model, leveraging a vast database of chemical reactions to predict feasible synthetic routes.
One-Step Synthesis Focus: Specifically designed for one-step synthesis, it provides concise and direct routes for your target compounds, streamlining the synthesis process.
Accurate Predictions: Utilizing the extensive PISTACHIO, BKMS_METABOLIC, PISTACHIO_RINGBREAKER, REAXYS, REAXYS_BIOCATALYSIS database, our tool offers high-accuracy predictions, reflecting the latest in chemical research and data.
Strategy Settings
Precursor scoring | Relevance Heuristic |
---|---|
Min. plausibility | 0.01 |
Model | Template_relevance |
Template Set | Pistachio/Bkms_metabolic/Pistachio_ringbreaker/Reaxys/Reaxys_biocatalysis |
Top-N result to add to graph | 6 |
Feasible Synthetic Routes
Q & A
Q1: What is the structural significance of chlorine substitution in 6,9-Dichloro-2-methoxyacridine?
A1: The presence of chlorine atoms, particularly at the C(9) position, significantly influences the molecular structure of 6,9-Dichloro-2-methoxyacridine. X-ray crystallography studies revealed that the addition of a chlorine atom at C(9) leads to a noticeable shortening of adjacent bonds compared to acridines with chlorine substitution solely at the C(6) position []. This structural modification can impact the molecule's interactions with biological targets.
Q2: How does the fluorescence of 6,9-Dichloro-2-methoxyacridine derivatives change upon binding to proteins?
A2: Researchers synthesized derivatives of 6,9-Dichloro-2-methoxyacridine, such as 9-(4′-Carboxyanilino)-6-chloro-2-methoxyacridine, to investigate their potential as fluorescence probes for drug-protein binding studies []. Interestingly, the fluorescence intensity of these derivatives, specifically 9-(4′-Carboxyanilino)-6-chloro-2-methoxyacridine, increased substantially upon binding to bovine serum albumin. This significant increase in fluorescence upon protein binding highlights the potential of these derivatives as valuable tools for studying drug-protein interactions.
Q3: Can 6,9-Dichloro-2-methoxyacridine be used as a building block for more complex molecules with potential biological activities?
A3: Yes, 6,9-Dichloro-2-methoxyacridine serves as a versatile starting material for synthesizing various derivatives with potential biological applications. One study successfully utilized 6,9-Dichloro-2-methoxyacridine to create dimeric acridine-derived molecules designed to intercalate into nucleic acids []. These dimeric structures demonstrated potent antiviral activity against MS2 phages, highlighting the potential of using 6,9-Dichloro-2-methoxyacridine as a scaffold for developing novel antiviral agents.
Q4: How does the stereochemistry of 6-chloro-9-(4'-diethylamino-1'-methylbutyl)-amino-2-methoxyacridine (quinacrine) influence its properties?
A5: Researchers successfully determined the absolute configuration of 6-chloro-9-(4'-diethylamino-1'-methylbutyl)-amino-2-methoxyacridine (quinacrine) enantiomers []. They demonstrated that each enantiomer, (−)-(R)-quinacrine and (+)−(S)-quinacrine, originates from the corresponding enantiomeric diamine precursor. This finding highlights the importance of stereochemistry in drug development, as different enantiomers can exhibit distinct biological activities and pharmacological profiles.
Disclaimer and Information on In-Vitro Research Products
Please be aware that all articles and product information presented on BenchChem are intended solely for informational purposes. The products available for purchase on BenchChem are specifically designed for in-vitro studies, which are conducted outside of living organisms. In-vitro studies, derived from the Latin term "in glass," involve experiments performed in controlled laboratory settings using cells or tissues. It is important to note that these products are not categorized as medicines or drugs, and they have not received approval from the FDA for the prevention, treatment, or cure of any medical condition, ailment, or disease. We must emphasize that any form of bodily introduction of these products into humans or animals is strictly prohibited by law. It is essential to adhere to these guidelines to ensure compliance with legal and ethical standards in research and experimentation.