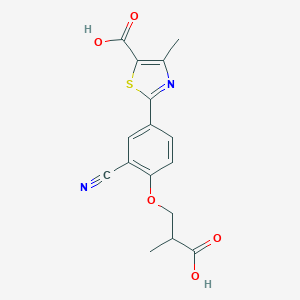
Febuxostat (67m-4)
Overview
Description
Febuxostat, also known as 2-(3-cyano-4-isobutoxyphenyl)-4-methylthiazole-5-carboxylic acid, is a medication primarily used to treat chronic gout and hyperuricemia. It is a non-purine selective inhibitor of xanthine oxidase, an enzyme responsible for the production of uric acid in the body. Febuxostat is particularly recommended for patients who cannot tolerate allopurinol, another xanthine oxidase inhibitor .
Mechanism of Action
Target of Action
Febuxostat primarily targets xanthine oxidase (XO) , an enzyme responsible for the synthesis of uric acid . This enzyme plays a crucial role in the metabolism of purines, which are vital components of nucleic acids .
Mode of Action
Febuxostat is a non-purine selective inhibitor of xanthine oxidase . It works by non-competitively blocking the active site of xanthine oxidase that contains a molybdenum pterin center . By inhibiting the activity of this enzyme, febuxostat reduces the production of uric acid, thereby decreasing serum uric acid levels .
Biochemical Pathways
The primary biochemical pathway affected by febuxostat is the purine degradation pathway . By inhibiting xanthine oxidase, febuxostat prevents the oxidation of hypoxanthine to xanthine and further oxidation to uric acid . This results in a decrease in uric acid production and a reduction in serum uric acid levels .
Pharmacokinetics
Febuxostat exhibits favorable pharmacokinetic properties. After oral administration, it has an oral availability of about 85% . The time course of plasma concentrations follows a two-compartment model with an initial half-life of approximately 2 hours and a terminal half-life of 9.4 ± 4.9 hours at daily doses of 40 mg or more . Febuxostat is extensively metabolized by oxidation (approximately 35%) and acyl glucuronidation (up to 40%); febuxostat acyl glucuronides are cleared by the kidney .
Result of Action
The primary molecular effect of febuxostat’s action is the reduction of serum uric acid levels . On a cellular level, febuxostat has been shown to alleviate MPP±induced inflammatory responses in astrocytes by reducing the expressions of IL-8, IL-1β, TNF-α, GFAP, MMP-2, and MMP-9 . It also mitigates the activation of the JNK/NF-κB signaling pathway by inhibiting the phosphorylation of JNK and nuclear translocation of NF-κB p65 .
Action Environment
The action of febuxostat can be influenced by various environmental factors. Furthermore, the effectiveness of febuxostat can be influenced by individual characteristics, requiring personalized dosing strategies .
Biochemical Analysis
Biochemical Properties
Febuxostat (67m-4) works by inhibiting the activity of an enzyme called xanthine oxidase, which is responsible for the synthesis of uric acid . This results in a decrease in serum uric acid levels . Febuxostat (67m-4) is extensively metabolised by oxidation (approximately 35%) and acyl glucuronidation (up to 40%); febuxostat acyl glucuronides are cleared by the kidney .
Cellular Effects
Febuxostat (67m-4) has been shown to have significant effects on various types of cells and cellular processes. For instance, it has been found to lower serum uric acid levels more potently than allopurinol while having minimal effects on other enzymes associated with purine and pyrimidine metabolism . It also suppresses the endoplasmic reticulum stress caused by various stress inducers through upregulation of SIRT1-AMPK-HO-1/thioredoxin expression .
Molecular Mechanism
Febuxostat (67m-4) exerts its effects at the molecular level by non-competitively blocking the molybdenum pterin center, which is the active site of xanthine oxidase . This inhibition of xanthine oxidase leads to a decrease in the production of uric acid .
Temporal Effects in Laboratory Settings
In a phase I, dose-escalation study, febuxostat (67m-4) was administered once daily on day 1, and days 3–14 . The pharmacokinetics of febuxostat were not time-dependent (day 14 vs day 1) and remained linear within the 10–120mg dose range . There is no significant accumulation of the drug during multiple dose administration .
Dosage Effects in Animal Models
In an animal model of metabolic syndrome, febuxostat was found to be mildly superior to allopurinol in lowering serum fasting glucose, lipids, uric acid, and antioxidant enzyme activities .
Metabolic Pathways
Febuxostat (67m-4) is involved in the metabolic pathway of uric acid production. It inhibits xanthine oxidase, an enzyme involved in the conversion of hypoxanthine to xanthine to uric acid . Febuxostat is extensively metabolised by oxidation and acyl glucuronidation .
Transport and Distribution
The apparent steady-state volume of distribution (V ss/F) of febuxostat ranges from 29 to 75 L, indicating a wide distribution within the body . It is extensively metabolised, and its metabolites are cleared by the kidney .
Preparation Methods
Synthetic Routes and Reaction Conditions
The synthesis of febuxostat involves several key steps:
Starting Materials: The synthesis begins with 4-hydroxybenzonitrile and thioacetamide.
Intermediate Formation: These starting materials react in hydrochloric acid solution to form 4-hydroxythiobenzamide.
Further Reactions: 4-hydroxythiobenzamide reacts with 2-chloroacetoacetic acid ethyl ester to produce 2-(4-hydroxyphenyl)-4-methylthiazole-5-carboxylic acid ethyl ester.
Formylation: The intermediate undergoes formylation with hexamine in a mixed acid system of methanesulfonic acid and trifluoroacetic acid to yield 2-(3-formyl-4-hydroxyphenyl)-4-methylthiazole-5-carboxylic acid ethyl ester.
Final Steps: The compound is then reacted with hydroxylamine hydrochloride, potassium carbonate, and iso-butyl bromide in a polar protonic solvent to form 2-(3-nitrile-4-isobutoxyphenyl)-4-methylthiazole-5-carboxylic acid ethyl ester.
Industrial Production Methods
Industrial production of febuxostat follows similar synthetic routes but on a larger scale. The process involves optimizing reaction conditions to ensure high yield and purity. The use of continuous flow reactors and advanced purification techniques like crystallization and chromatography are common in industrial settings to achieve the desired product quality.
Chemical Reactions Analysis
Types of Reactions
Febuxostat undergoes various chemical reactions, including:
Oxidation: Febuxostat can be oxidized to form different metabolites.
Reduction: Reduction reactions can modify the nitrile group in febuxostat.
Substitution: Substitution reactions can occur at the aromatic ring or the thiazole ring.
Common Reagents and Conditions
Oxidation: Common oxidizing agents include hydrogen peroxide and potassium permanganate.
Reduction: Reducing agents like lithium aluminum hydride or sodium borohydride are used.
Substitution: Halogenating agents like bromine or chlorine can be used for substitution reactions.
Major Products
The major products formed from these reactions include various oxidized and reduced metabolites, as well as substituted derivatives of febuxostat.
Scientific Research Applications
Febuxostat has a wide range of scientific research applications:
Chemistry: It is used as a model compound to study xanthine oxidase inhibition and its effects on uric acid production.
Biology: Febuxostat is used in biological studies to understand its impact on cellular metabolism and enzyme activity.
Medicine: Clinically, febuxostat is used to manage chronic gout and hyperuricemia. .
Industry: In the pharmaceutical industry, febuxostat is a key ingredient in medications for gout management.
Comparison with Similar Compounds
Similar Compounds
Allopurinol: Another xanthine oxidase inhibitor used to treat gout and hyperuricemia.
Topiroxostat: A newer xanthine oxidase inhibitor with similar applications.
Uniqueness of Febuxostat
Selectivity: Febuxostat is more selective for xanthine oxidase compared to allopurinol, which also affects other enzymes involved in purine metabolism.
Efficacy: Clinical studies have shown that febuxostat is more effective than standard doses of allopurinol in lowering serum uric acid levels
Safety Profile: Febuxostat has a different safety profile compared to allopurinol, with fewer hypersensitivity reactions but a higher risk of cardiovascular events.
Febuxostat stands out due to its high selectivity and efficacy in reducing uric acid levels, making it a valuable option for patients who cannot tolerate other treatments.
Biological Activity
Febuxostat, also known as 67M-4, is a selective xanthine oxidase inhibitor primarily used for the management of hyperuricemia and chronic gout. Recent studies have expanded its profile to include antibacterial properties, particularly against Mycobacterium tuberculosis (Mtb). This article reviews the biological activity of febuxostat, focusing on its pharmacodynamics, efficacy in treating gout and hyperuricemia, and emerging antibacterial effects.
Febuxostat operates by inhibiting xanthine oxidase (XOR), an enzyme involved in the conversion of hypoxanthine to xanthine and subsequently to uric acid. This inhibition leads to a significant reduction in serum urate levels. Unlike allopurinol, febuxostat binds more effectively to both the oxidized and reduced forms of XOR, resulting in a more stable enzyme-inhibitor complex .
Key Mechanisms:
- Selective Inhibition : Febuxostat selectively inhibits XOR and xanthine dehydrogenase, which helps reduce oxidative stress by decreasing reactive oxygen species (ROS) production .
- Metabolism : Febuxostat is metabolized in the liver through UDP-glucuronosyltransferase and cytochrome P450 enzymes, producing active metabolites 67M-1, 67M-2, and 67M-4 that also contribute to its therapeutic effects .
Efficacy in Gout Management
Clinical trials have demonstrated febuxostat's efficacy in lowering serum urate levels in patients with hyperuricemia and gout. A review of multiple studies shows that febuxostat effectively maintains serum urate levels below 6 mg/dL, with a favorable safety profile compared to allopurinol .
Clinical Findings:
- Dosage and Results : In a five-year study involving 116 subjects, 93% maintained serum urate levels below 6.0 mg/dL with various dosages of febuxostat (40-120 mg daily). The study also noted significant reductions in gout flares and resolution of tophi in many patients .
- Adverse Effects : The most common side effects included liver function abnormalities, gastrointestinal symptoms, and headaches. However, these were generally mild and transient .
Antibacterial Activity Against Mycobacterium tuberculosis
Recent research has uncovered febuxostat's potential as an antibacterial agent against Mtb. Studies indicate that febuxostat significantly inhibits mycobacterial growth in vitro and in vivo.
Key Findings:
- In Vitro Studies : Febuxostat demonstrated a concentration-dependent bactericidal effect on Mtb with minimal impact on host cell viability. The minimum inhibitory concentration (MIC) for effective bacterial growth suppression was established through various assays .
- In Vivo Efficacy : In murine models of tuberculosis, febuxostat treatment resulted in reduced bacterial loads in the lungs and spleen without exacerbating lung inflammation. This suggests that febuxostat may be a promising candidate for tuberculosis treatment, particularly given the current challenges in antibiotic resistance .
Summary of Research Findings
The following table summarizes key research findings related to febuxostat's biological activity:
Properties
IUPAC Name |
2-[4-(2-carboxypropoxy)-3-cyanophenyl]-4-methyl-1,3-thiazole-5-carboxylic acid | |
---|---|---|
Source | PubChem | |
URL | https://pubchem.ncbi.nlm.nih.gov | |
Description | Data deposited in or computed by PubChem | |
InChI |
InChI=1S/C16H14N2O5S/c1-8(15(19)20)7-23-12-4-3-10(5-11(12)6-17)14-18-9(2)13(24-14)16(21)22/h3-5,8H,7H2,1-2H3,(H,19,20)(H,21,22) | |
Source | PubChem | |
URL | https://pubchem.ncbi.nlm.nih.gov | |
Description | Data deposited in or computed by PubChem | |
InChI Key |
LCBACLAPKJRMIF-UHFFFAOYSA-N | |
Source | PubChem | |
URL | https://pubchem.ncbi.nlm.nih.gov | |
Description | Data deposited in or computed by PubChem | |
Canonical SMILES |
CC1=C(SC(=N1)C2=CC(=C(C=C2)OCC(C)C(=O)O)C#N)C(=O)O | |
Source | PubChem | |
URL | https://pubchem.ncbi.nlm.nih.gov | |
Description | Data deposited in or computed by PubChem | |
Molecular Formula |
C16H14N2O5S | |
Source | PubChem | |
URL | https://pubchem.ncbi.nlm.nih.gov | |
Description | Data deposited in or computed by PubChem | |
DSSTOX Substance ID |
DTXSID701148050 | |
Record name | 2-[4-(2-Carboxypropoxy)-3-cyanophenyl]-4-methyl-5-thiazolecarboxylic acid | |
Source | EPA DSSTox | |
URL | https://comptox.epa.gov/dashboard/DTXSID701148050 | |
Description | DSSTox provides a high quality public chemistry resource for supporting improved predictive toxicology. | |
Molecular Weight |
346.4 g/mol | |
Source | PubChem | |
URL | https://pubchem.ncbi.nlm.nih.gov | |
Description | Data deposited in or computed by PubChem | |
CAS No. |
407582-49-4 | |
Record name | 2-[4-(2-Carboxypropoxy)-3-cyanophenyl]-4-methyl-5-thiazolecarboxylic acid | |
Source | CAS Common Chemistry | |
URL | https://commonchemistry.cas.org/detail?cas_rn=407582-49-4 | |
Description | CAS Common Chemistry is an open community resource for accessing chemical information. Nearly 500,000 chemical substances from CAS REGISTRY cover areas of community interest, including common and frequently regulated chemicals, and those relevant to high school and undergraduate chemistry classes. This chemical information, curated by our expert scientists, is provided in alignment with our mission as a division of the American Chemical Society. | |
Explanation | The data from CAS Common Chemistry is provided under a CC-BY-NC 4.0 license, unless otherwise stated. | |
Record name | Febuxostat (67m-4) | |
Source | ChemIDplus | |
URL | https://pubchem.ncbi.nlm.nih.gov/substance/?source=chemidplus&sourceid=0407582494 | |
Description | ChemIDplus is a free, web search system that provides access to the structure and nomenclature authority files used for the identification of chemical substances cited in National Library of Medicine (NLM) databases, including the TOXNET system. | |
Record name | 2-[4-(2-Carboxypropoxy)-3-cyanophenyl]-4-methyl-5-thiazolecarboxylic acid | |
Source | EPA DSSTox | |
URL | https://comptox.epa.gov/dashboard/DTXSID701148050 | |
Description | DSSTox provides a high quality public chemistry resource for supporting improved predictive toxicology. | |
Record name | 2-(4-(2-CARBOXYPROPOXY)-3-CYANOPHENYL)-4-METHYL-5-THIAZOLECARBOXYLIC ACID | |
Source | FDA Global Substance Registration System (GSRS) | |
URL | https://gsrs.ncats.nih.gov/ginas/app/beta/substances/NRN27Y63ZG | |
Description | The FDA Global Substance Registration System (GSRS) enables the efficient and accurate exchange of information on what substances are in regulated products. Instead of relying on names, which vary across regulatory domains, countries, and regions, the GSRS knowledge base makes it possible for substances to be defined by standardized, scientific descriptions. | |
Explanation | Unless otherwise noted, the contents of the FDA website (www.fda.gov), both text and graphics, are not copyrighted. They are in the public domain and may be republished, reprinted and otherwise used freely by anyone without the need to obtain permission from FDA. Credit to the U.S. Food and Drug Administration as the source is appreciated but not required. | |
Retrosynthesis Analysis
AI-Powered Synthesis Planning: Our tool employs the Template_relevance Pistachio, Template_relevance Bkms_metabolic, Template_relevance Pistachio_ringbreaker, Template_relevance Reaxys, Template_relevance Reaxys_biocatalysis model, leveraging a vast database of chemical reactions to predict feasible synthetic routes.
One-Step Synthesis Focus: Specifically designed for one-step synthesis, it provides concise and direct routes for your target compounds, streamlining the synthesis process.
Accurate Predictions: Utilizing the extensive PISTACHIO, BKMS_METABOLIC, PISTACHIO_RINGBREAKER, REAXYS, REAXYS_BIOCATALYSIS database, our tool offers high-accuracy predictions, reflecting the latest in chemical research and data.
Strategy Settings
Precursor scoring | Relevance Heuristic |
---|---|
Min. plausibility | 0.01 |
Model | Template_relevance |
Template Set | Pistachio/Bkms_metabolic/Pistachio_ringbreaker/Reaxys/Reaxys_biocatalysis |
Top-N result to add to graph | 6 |
Feasible Synthetic Routes
Disclaimer and Information on In-Vitro Research Products
Please be aware that all articles and product information presented on BenchChem are intended solely for informational purposes. The products available for purchase on BenchChem are specifically designed for in-vitro studies, which are conducted outside of living organisms. In-vitro studies, derived from the Latin term "in glass," involve experiments performed in controlled laboratory settings using cells or tissues. It is important to note that these products are not categorized as medicines or drugs, and they have not received approval from the FDA for the prevention, treatment, or cure of any medical condition, ailment, or disease. We must emphasize that any form of bodily introduction of these products into humans or animals is strictly prohibited by law. It is essential to adhere to these guidelines to ensure compliance with legal and ethical standards in research and experimentation.