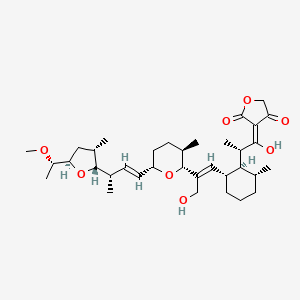
Tetronasin
- Click on QUICK INQUIRY to receive a quote from our team of experts.
- With the quality product at a COMPETITIVE price, you can focus more on your research.
Overview
Description
Tetronasin 5930 is a polyether tetronate ionophore antibiotic. It is produced by the terrestrial Gram-positive bacterium Actinomadura verrucosospora. This compound 5930 is characterized by containing a tetronate, cyclohexane, tetrahydropyran, and at least one tetrahydrofuran ring . This compound is known for its high affinity to calcium ions and is used as a supplement in the diet for cattle to improve feed efficiency .
Preparation Methods
Synthetic Routes and Reaction Conditions: The biosynthesis of tetronasin involves the formation of a polyketide-derived backbone, which is then modified by various enzymatic reactions. The biosynthetic pathway includes the incorporation of a glycerol-derived three-carbon unit into the tetronic acid moiety, followed by the formation of mature aglycone via a Diels-Alder-like reaction . The preparation of deuterium-labeled tri- and tetraketides as putative biosynthetic precursors of this compound has been studied extensively .
Industrial Production Methods: Industrial production of this compound typically involves fermentation processes using Actinomadura verrucosospora. The fermentation conditions are optimized to maximize the yield of this compound. The biosynthetic gene cluster responsible for this compound production has been identified, allowing for genetic manipulation to enhance production .
Chemical Reactions Analysis
Types of Reactions: Tetronasin undergoes various chemical reactions, including oxidation, reduction, and substitution reactions. Fragmentation studies using electrospray ionization mass spectrometry have shown that this compound primarily undergoes fragmentation via the loss of water, leading to the formation of a carbocation .
Common Reagents and Conditions: Common reagents used in the reactions of this compound include oxidizing agents such as potassium permanganate and reducing agents like sodium borohydride. The reactions are typically carried out under mild conditions to prevent degradation of the compound .
Major Products Formed: The major products formed from the reactions of this compound include various oxidized and reduced derivatives. These derivatives retain the core structure of this compound but exhibit different biological activities .
Scientific Research Applications
Tetronasin has a wide range of scientific research applications In chemistry, it is used as a model compound to study ionophore antibiotics and their interactions with metal ions In biology, this compound is used to investigate the mechanisms of ion transport across cell membranesIn the industry, this compound is used as a feed additive to improve the efficiency of cattle production .
Mechanism of Action
Tetronasin exerts its effects by forming stable complexes with metal ions, particularly calcium ions. The oxygen atoms in the tetrahydropyran and tetrahydrofuran rings serve as ligands for the complexation of these ions. The resulting complexes facilitate the transport of metal ions across cell membranes, disrupting ion gradients and leading to the death of target organisms .
Comparison with Similar Compounds
Tetronasin is similar to other polyether tetronate antibiotics such as tetromadurin and tetronomycin. These compounds share a common structural motif, including a tetronate moiety and multiple ether rings. this compound is unique in its high affinity for calcium ions and its specific use as a feed additive for cattle .
List of Similar Compounds:- Tetromadurin
- Tetronomycin
- Tetrocarcin A
- Kijanimicin
- Chlorothricin
This compound 5930 stands out due to its specific applications and unique structural features, making it a valuable compound in various fields of research and industry.
Properties
CAS No. |
75139-06-9 |
---|---|
Molecular Formula |
C35H54O8 |
Molecular Weight |
602.8 g/mol |
IUPAC Name |
(3E)-3-[(2S)-1-hydroxy-2-[(1S,2S,6R)-2-[(E)-3-hydroxy-2-[(2R,3R,6S)-6-[(E,3S)-3-[(2R,3S,5R)-5-[(1S)-1-methoxyethyl]-3-methyloxolan-2-yl]but-1-enyl]-3-methyloxan-2-yl]prop-1-enyl]-6-methylcyclohexyl]propylidene]oxolane-2,4-dione |
InChI |
InChI=1S/C35H54O8/c1-19-9-8-10-25(30(19)23(5)32(38)31-28(37)18-41-35(31)39)16-26(17-36)34-21(3)12-14-27(42-34)13-11-20(2)33-22(4)15-29(43-33)24(6)40-7/h11,13,16,19-25,27,29-30,33-34,36,38H,8-10,12,14-15,17-18H2,1-7H3/b13-11+,26-16+,32-31+/t19-,20+,21-,22+,23+,24+,25-,27-,29-,30-,33+,34-/m1/s1 |
InChI Key |
XZJAKURZQBNKKX-QFQDJZPHSA-N |
Isomeric SMILES |
C[C@@H]1CCC[C@@H]([C@H]1[C@H](C)/C(=C\2/C(=O)COC2=O)/O)/C=C(\CO)/[C@H]3[C@@H](CC[C@H](O3)/C=C/[C@H](C)[C@H]4[C@H](C[C@@H](O4)[C@H](C)OC)C)C |
Canonical SMILES |
CC1CCCC(C1C(C)C(=C2C(=O)COC2=O)O)C=C(CO)C3C(CCC(O3)C=CC(C)C4C(CC(O4)C(C)OC)C)C |
Origin of Product |
United States |
Disclaimer and Information on In-Vitro Research Products
Please be aware that all articles and product information presented on BenchChem are intended solely for informational purposes. The products available for purchase on BenchChem are specifically designed for in-vitro studies, which are conducted outside of living organisms. In-vitro studies, derived from the Latin term "in glass," involve experiments performed in controlled laboratory settings using cells or tissues. It is important to note that these products are not categorized as medicines or drugs, and they have not received approval from the FDA for the prevention, treatment, or cure of any medical condition, ailment, or disease. We must emphasize that any form of bodily introduction of these products into humans or animals is strictly prohibited by law. It is essential to adhere to these guidelines to ensure compliance with legal and ethical standards in research and experimentation.