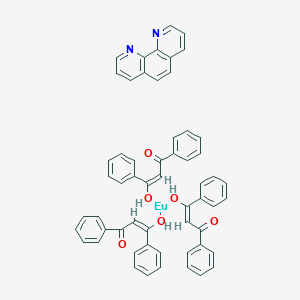
Tris(dibenzoylmethane)mono(phenanthroline)europium (III)
Overview
Description
Tris(dibenzoylmethane)mono(phenanthroline)europium (III) is a coordination compound that features europium as the central metal ion. This compound is known for its luminescent properties, making it valuable in various scientific and industrial applications. The compound is often used in the development of organic light-emitting diodes (OLEDs) and other optoelectronic devices due to its ability to emit red light efficiently .
Mechanism of Action
Target of Action
Tris(dibenzoylmethane)mono(phenanthroline)europium (III) is primarily used as a light-emitting compound in organic light-emitting diodes (OLEDs) . Its primary targets are the dye molecules introduced in the form of an emission layer on the hole transporting side .
Mode of Action
The compound interacts with its targets by being excited by a blue light-emitting diode containing a hole blocking layer (HBL) .
Biochemical Pathways
The compound affects the light emission pathway in OLEDs. When excited, it emits light, contributing to the overall luminance output of the device .
Pharmacokinetics
For instance, the luminance output of a device using this compound was improved by partially adding a hole transport material to the emitting layer, increasing the penetration of holes into the emission region .
Result of Action
The result of the compound’s action is the production of pure red light in OLEDs . A maximum luminance output of 200 cd/m^2 at 15 V was achieved without optimizing the layer thickness .
Action Environment
The action of Tris(dibenzoylmethane)mono(phenanthroline)europium (III) can be influenced by environmental factors such as temperature. For example, temperature-sensitive europium complexes of this compound may be used to fabricate a dual optical sensor to detect oxygen and any change in temperature .
Preparation Methods
Synthetic Routes and Reaction Conditions
The synthesis of Tris(dibenzoylmethane)mono(phenanthroline)europium (III) typically involves the reaction of europium chloride with dibenzoylmethane and phenanthroline in an appropriate solvent. The reaction is usually carried out under reflux conditions to ensure complete reaction and formation of the desired complex .
Industrial Production Methods
While specific industrial production methods are not extensively documented, the synthesis generally follows the same principles as laboratory preparation but on a larger scale. This involves scaling up the reaction while maintaining the necessary conditions to ensure high yield and purity of the compound .
Chemical Reactions Analysis
Types of Reactions
Tris(dibenzoylmethane)mono(phenanthroline)europium (III) primarily undergoes coordination reactions due to the presence of the europium ion. It can also participate in substitution reactions where ligands can be replaced by other coordinating molecules .
Common Reagents and Conditions
Common reagents used in reactions involving this compound include solvents like ethanol or methanol, and other coordinating ligands that can replace dibenzoylmethane or phenanthroline under specific conditions .
Major Products Formed
The major products formed from reactions involving Tris(dibenzoylmethane)mono(phenanthroline)europium (III) depend on the nature of the substituting ligands. For example, replacing phenanthroline with another ligand can result in a different europium complex with potentially altered luminescent properties .
Scientific Research Applications
Tris(dibenzoylmethane)mono(phenanthroline)europium (III) has a wide range of applications in scientific research:
Comparison with Similar Compounds
Similar Compounds
Tris(dibenzoylmethane)mono(5-amino-1,10-phenanthroline)europium (III): Similar in structure but with an amino group on the phenanthroline ligand, which can alter its luminescent properties.
Europium(III) tris(1,3-diphenyl-1,3-propanedionato) mono(1,10-phenanthroline): Another similar compound with slight variations in the ligand structure.
Uniqueness
Tris(dibenzoylmethane)mono(phenanthroline)europium (III) is unique due to its specific combination of ligands, which provide a balance of stability and luminescent efficiency. This makes it particularly suitable for applications in optoelectronics and bioimaging .
Properties
IUPAC Name |
europium;(E)-3-hydroxy-1,3-diphenylprop-2-en-1-one;1,10-phenanthroline | |
---|---|---|
Source | PubChem | |
URL | https://pubchem.ncbi.nlm.nih.gov | |
Description | Data deposited in or computed by PubChem | |
InChI |
InChI=1S/3C15H12O2.C12H8N2.Eu/c3*16-14(12-7-3-1-4-8-12)11-15(17)13-9-5-2-6-10-13;1-3-9-5-6-10-4-2-8-14-12(10)11(9)13-7-1;/h3*1-11,16H;1-8H;/b3*14-11+;; | |
Source | PubChem | |
URL | https://pubchem.ncbi.nlm.nih.gov | |
Description | Data deposited in or computed by PubChem | |
InChI Key |
DYKOLWWJTALFFU-RWBKAWJDSA-N | |
Source | PubChem | |
URL | https://pubchem.ncbi.nlm.nih.gov | |
Description | Data deposited in or computed by PubChem | |
Canonical SMILES |
C1=CC=C(C=C1)C(=CC(=O)C2=CC=CC=C2)O.C1=CC=C(C=C1)C(=CC(=O)C2=CC=CC=C2)O.C1=CC=C(C=C1)C(=CC(=O)C2=CC=CC=C2)O.C1=CC2=C(C3=C(C=CC=N3)C=C2)N=C1.[Eu] | |
Source | PubChem | |
URL | https://pubchem.ncbi.nlm.nih.gov | |
Description | Data deposited in or computed by PubChem | |
Isomeric SMILES |
C1=CC=C(C=C1)/C(=C\C(=O)C2=CC=CC=C2)/O.C1=CC=C(C=C1)/C(=C\C(=O)C2=CC=CC=C2)/O.C1=CC=C(C=C1)/C(=C\C(=O)C2=CC=CC=C2)/O.C1=CC2=C(N=C1)C3=C(C=C2)C=CC=N3.[Eu] | |
Source | PubChem | |
URL | https://pubchem.ncbi.nlm.nih.gov | |
Description | Data deposited in or computed by PubChem | |
Molecular Formula |
C57H44EuN2O6 | |
Source | PubChem | |
URL | https://pubchem.ncbi.nlm.nih.gov | |
Description | Data deposited in or computed by PubChem | |
Molecular Weight |
1004.9 g/mol | |
Source | PubChem | |
URL | https://pubchem.ncbi.nlm.nih.gov | |
Description | Data deposited in or computed by PubChem | |
Retrosynthesis Analysis
AI-Powered Synthesis Planning: Our tool employs the Template_relevance Pistachio, Template_relevance Bkms_metabolic, Template_relevance Pistachio_ringbreaker, Template_relevance Reaxys, Template_relevance Reaxys_biocatalysis model, leveraging a vast database of chemical reactions to predict feasible synthetic routes.
One-Step Synthesis Focus: Specifically designed for one-step synthesis, it provides concise and direct routes for your target compounds, streamlining the synthesis process.
Accurate Predictions: Utilizing the extensive PISTACHIO, BKMS_METABOLIC, PISTACHIO_RINGBREAKER, REAXYS, REAXYS_BIOCATALYSIS database, our tool offers high-accuracy predictions, reflecting the latest in chemical research and data.
Strategy Settings
Precursor scoring | Relevance Heuristic |
---|---|
Min. plausibility | 0.01 |
Model | Template_relevance |
Template Set | Pistachio/Bkms_metabolic/Pistachio_ringbreaker/Reaxys/Reaxys_biocatalysis |
Top-N result to add to graph | 6 |
Feasible Synthetic Routes
Disclaimer and Information on In-Vitro Research Products
Please be aware that all articles and product information presented on BenchChem are intended solely for informational purposes. The products available for purchase on BenchChem are specifically designed for in-vitro studies, which are conducted outside of living organisms. In-vitro studies, derived from the Latin term "in glass," involve experiments performed in controlled laboratory settings using cells or tissues. It is important to note that these products are not categorized as medicines or drugs, and they have not received approval from the FDA for the prevention, treatment, or cure of any medical condition, ailment, or disease. We must emphasize that any form of bodily introduction of these products into humans or animals is strictly prohibited by law. It is essential to adhere to these guidelines to ensure compliance with legal and ethical standards in research and experimentation.