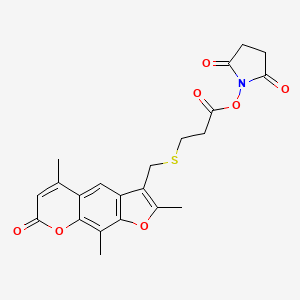
Amt-nhs
- Click on QUICK INQUIRY to receive a quote from our team of experts.
- With the quality product at a COMPETITIVE price, you can focus more on your research.
Overview
Description
AMT-NHS (4′-Aminomethyltrioxsalen-N-hydroxysuccinimide ester) is a bifunctional chemical crosslinker designed to study RNA-protein interactions in living cells. It combines a photoreactive AMT moiety—a derivative of the psoralen family—with an NHS ester group, enabling covalent bonding with RNA nucleobases (via intercalation and UV activation) and lysine residues on proteins . Synthesized through a three-step reaction, this compound exhibits cell permeability, allowing it to penetrate live yeast cells and crosslink RNA-protein complexes in vivo .
Its primary application lies in capturing interactions between RNA-binding proteins (e.g., Cbf5 in H/ACA snoRNP complexes) and structured RNA regions, including single-stranded and double-stranded motifs .
Preparation Methods
Synthetic Routes and Reaction Conditions
The synthesis of AMT-NHS involves the reaction of 4’-aminomethyltrioxsalen with N-hydroxysuccinimide in the presence of a coupling agent such as N,N’-dicyclohexylcarbodiimide (DCC). The reaction typically occurs in an organic solvent like dimethylformamide (DMF) under mild conditions .
Industrial Production Methods
Industrial production of this compound follows similar synthetic routes but on a larger scale. The process involves careful control of reaction conditions to ensure high yield and purity. The use of automated reactors and purification systems helps in scaling up the production while maintaining consistency.
Chemical Reactions Analysis
Types of Reactions
AMT-NHS undergoes several types of chemical reactions, including:
Substitution Reactions: The N-hydroxysuccinimide ester group reacts with primary amines to form stable amide bonds.
Photoreactions: The psoralen derivative can intercalate into RNA helices and react with pyrimidines upon photo-activation.
Common Reagents and Conditions
Primary Amines: React with the N-hydroxysuccinimide ester group under slightly alkaline conditions (pH 7.2-8.5) to form amide bonds.
UV Light: Activates the psoralen derivative to form crosslinks with RNA bases.
Major Products Formed
Amide Bonds: Formed between the N-hydroxysuccinimide ester group and primary amines.
RNA-Protein Crosslinks: Formed upon photo-activation of the psoralen derivative.
Scientific Research Applications
Key Applications
-
Mapping RNA-Protein Interactions
- AMT-NHS has been successfully used to map RNA-protein interactions within living cells. A study demonstrated its ability to crosslink Cbf5 (a protein) with H/ACA small nucleolar RNAs (snoRNAs) in yeast cells, showcasing its specificity and efficiency compared to traditional UV crosslinking methods .
-
Characterization of Crosslinking Patterns
- The crosslinking patterns induced by this compound differ significantly from those produced by UV light. While UV requires zero-distance interactions, this compound can capture more diverse RNA structures, including both single-stranded and double-stranded regions . This capability allows researchers to investigate interactions that are otherwise difficult to detect.
- Applications in Drug Discovery
Comparative Data on Crosslinking Methods
Feature | This compound | UV Crosslinking |
---|---|---|
Crosslinking Efficiency | Moderate; specific to certain residues | High; requires zero-distance contact |
Targeted Regions | Single and double-stranded RNAs | Primarily single-stranded RNAs |
Application Context | In vivo and in vitro | Mostly in vitro |
Interaction Diversity | Captures diverse RNA structures | Limited to specific structures |
Case Studies
Case Study 1: Crosslinking of Cbf5 with H/ACA snoRNAs
- In a detailed study, researchers utilized this compound to crosslink Cbf5 with H/ACA snoRNAs in both lysed and living yeast cells. The results indicated that this compound produced distinct crosslinking patterns compared to UV light, allowing for a more comprehensive mapping of RNA-protein interactions .
Case Study 2: Drug Interaction Mapping
- Another application involved using this compound to explore the interactions between various drugs and RBPs. By identifying enriched gene targets associated with these compounds, researchers could establish potential pathways for drug repurposing, highlighting the compound's versatility in biomedical research .
Mechanism of Action
AMT-NHS exerts its effects through two primary mechanisms:
Amide Bond Formation: The N-hydroxysuccinimide ester group reacts with primary amines to form stable amide bonds, facilitating the conjugation of biomolecules.
Photo-activated Crosslinking: The psoralen derivative intercalates into RNA helices and, upon exposure to UV light, forms covalent crosslinks with pyrimidine bases.
Comparison with Similar Compounds
AMT-NHS vs. UV Crosslinking
UV crosslinking is a widely used method for studying RNA-protein interactions but has critical limitations compared to this compound:
Key Findings :
- This compound induces distinct crosslinking patterns compared to UV, enabling the detection of interactions in structurally constrained regions (e.g., Cbf5 binding to the pseudouridylation pocket) .
This compound vs. Formaldehyde Crosslinking
Formaldehyde is another chemical crosslinker used for stabilizing RNA-protein complexes. However:
- Mechanism : Formaldehyde creates short-range (∼2 Å) crosslinks between primary amines and aromatic rings, limiting its utility for structured RNA-protein interfaces .
- Reversibility : Formaldehyde crosslinks are reversible under heat or acidic conditions, whereas this compound forms stable covalent bonds .
- Resolution : this compound provides higher spatial resolution due to its RNA intercalation-driven specificity, whereas formaldehyde crosslinking is less precise .
This compound vs. Biotinylated Crosslinkers
Biotin-based crosslinkers (e.g., psoralen-biotin) enable affinity purification but lack the NHS ester group for simultaneous protein targeting. This compound’s bifunctionality allows concurrent RNA-protein crosslinking without additional conjugation steps .
Limitations of this compound :
- Surface Accessibility : Only crosslinks RNA-protein complexes exposed on the ribonucleoprotein (RNP) surface .
- Temporal Resolution : Prolonged incubation (≥2 hours) may alter cellular RNA/protein dynamics .
- Sequence Bias : Preferential crosslinking with pyrimidines may underrepresent purine-rich interactions .
Biological Activity
AMT-NHS (N-hydroxysuccinimide ester of a psoralen derivative) is a crosslinking agent designed to facilitate the study of RNA-protein interactions. This compound has shown significant promise in various biological applications, particularly in mapping RNA-binding proteins in vivo and in vitro. This article will delve into the biological activity of this compound, highlighting its mechanisms, effectiveness, and relevant case studies.
This compound operates by forming covalent bonds between RNA bases and primary amines on proteins, enabling the capture of RNA-protein complexes. This crosslinking occurs through exposure to UV light, which activates the psoralen moiety of the compound. The specificity and efficiency of this compound have been demonstrated in several studies, showcasing its ability to penetrate living cells and facilitate crosslinking without the limitations associated with traditional UV crosslinking methods.
Comparative Analysis of Crosslinking Methods
The following table summarizes the differences between this compound and traditional UV crosslinking methods:
Feature | This compound | UV Crosslinking |
---|---|---|
Mechanism | Covalent bonding via psoralen | Direct UV-induced dimerization |
Specificity | High specificity for RNA-protein interactions | Variable specificity depending on RNA structure |
Crosslinking Patterns | Distinct patterns across single and double-stranded regions | Generally favors single-stranded regions |
In Vivo Application | Effective in living cells | Limited due to cell damage |
Efficiency | Moderate to high | High but can induce background noise |
Case Study 1: Mapping RNA-Protein Interactions
A study published in Nature Communications demonstrated that this compound could effectively map RNA-protein interactions in yeast cells. The researchers used this compound to crosslink Cbf5 (a protein) with H/ACA snoRNAs, revealing distinct crosslinking patterns compared to UV methods. The correlation coefficient for crosslinking efficiency between in vitro and in vivo conditions was found to be R=0.9, indicating a high level of consistency in results across different experimental setups .
Case Study 2: Crosslinking Efficiency
In another research effort, this compound was tested against various RNA structures to evaluate its crosslinking efficiency. The results indicated that this compound was able to target both single-stranded (approximately 73%) and double-stranded (approximately 27%) regions effectively, with a notable increase in double-stranded targeting compared to UV methods, which typically favored single-stranded regions . This versatility makes this compound a valuable tool for studying complex RNA structures.
Research Findings
Recent findings emphasize the following aspects of this compound's biological activity:
- Cell Penetration: this compound demonstrates excellent cell permeability, allowing for effective application in live-cell imaging and interaction studies .
- Crosslinking Patterns: The compound induces unique crosslinking patterns that differ significantly from those produced by UV light, suggesting that it can provide more nuanced insights into RNA-protein interactions .
- Applications in Disease Research: The ability to map RNA-protein interactions has implications for understanding diseases linked to RNA misregulation, such as cancer and neurodegenerative disorders .
Q & A
Basic Research Questions
Q. What experimental advantages does AMT-NHS offer over traditional methods like CLIP in studying RNA-protein interactions?
this compound combines 4'-aminomethyl trioxsalen (AMT) for RNA crosslinking and an NHS ester for covalent binding to protein amines. This design enables efficient capture of long-range RNA-protein interactions, which are challenging for conventional CLIP methods. Key advantages include:
- Higher crosslinking efficiency : Demonstrated through comparative immunoblotting and sequencing validation .
- Flexibility : The NHS ester reacts with lysine residues, expanding targetable protein regions .
- Compatibility with sequencing : Post-crosslinking RNA can be purified and analyzed via cDNA libraries, ensuring compatibility with next-generation sequencing workflows .
Q. How is this compound synthesized and characterized for use in crosslinking experiments?
this compound synthesis involves three steps:
- Synthesis of 3-(chloromethyl)-2,5,9-trimethyl-7H-furo[3,2-g]chromen-7-one (Compound 2) : Produced via trioxane and hydrochloric acid reactions .
- Thioether linkage formation : Compound 2 reacts with 3-mercaptopropionic acid in tetrahydrofuran (THF) to introduce a carboxylic acid group .
- NHS ester activation : The carboxylic acid is activated using N-hydroxysuccinimide (NHS) and dicyclohexylcarbodiimide (DCC) in dichloromethane . Characterization includes mass spectrometry, UV absorption profiling, and functional validation in crosslinking assays .
Q. What validation steps are essential to confirm this compound-mediated RNA-protein interactions?
Rigorous validation requires:
- Immunoprecipitation and immunoblotting : To verify target protein enrichment post-crosslinking .
- Parallel sample analysis : Replicate experiments ensure reproducibility, with sequencing data compared across batches .
- Comparison to established datasets : Overlap with known RNA-protein interaction databases (e.g., CLIP-seq) confirms method accuracy .
Q. How should researchers control for non-specific binding in this compound experiments?
Critical controls include:
- Untreated controls : Omit this compound to assess baseline protein-RNA associations .
- Protease digestion : Post-crosslinking treatment with proteinase K removes non-covalently bound proteins .
- Competitive inhibitors : Use excess free NHS ester to block non-specific lysine interactions .
Advanced Research Questions
Q. What methodological considerations are critical when integrating this compound-based crosslinking with next-generation sequencing to ensure data reliability?
Key considerations:
- RNA integrity : Use RNase inhibitors during crosslinking to prevent degradation .
- Library preparation bias : Normalize cDNA library sizes and employ spike-in controls (e.g., exogenous RNA) to quantify technical variability .
- Bioinformatics pipelines : Customize alignment tools to account for crosslinking-induced RNA modifications, such as truncated reads .
Q. How can researchers optimize this compound concentration and UV exposure to balance crosslinking efficiency and cellular toxicity?
Optimization strategies:
- Dose-response assays : Test this compound concentrations (e.g., 0.1–10 µM) and UV durations (10–60 seconds) to identify thresholds where crosslinking efficiency plateaus without reducing cell viability .
- Viability assays : Pair crosslinking experiments with ATP-based luminescence or trypan blue exclusion tests to quantify toxicity .
- Time-course studies : Shorter UV exposure (e.g., 20 seconds) often suffices for efficient crosslinking while minimizing DNA damage .
Q. How should researchers address discrepancies between this compound-derived data and traditional CLIP results?
Discrepancies may arise due to:
- Interaction range : this compound captures long-range interactions missed by CLIP. Validate using orthogonal methods like RIP-seq .
- Protein specificity : NHS ester targets lysine-rich regions, potentially biasing toward certain protein domains. Perform mutagenesis (e.g., lysine-to-arginine substitutions) to confirm binding sites .
- Data normalization : Use quantile normalization or DESeq2 to adjust for technical differences in sequencing depth .
Q. What strategies enhance the reproducibility of this compound experiments in complex biological systems (e.g., mammalian tissues)?
To improve reproducibility:
- Standardize lysis buffers : Include 1% SDS to solubilize membrane-bound complexes without disrupting crosslinks .
- Batch-effect mitigation : Process all samples for a single experiment in parallel, using the same reagent lots .
- Blinded analysis : Assign sample IDs randomly to avoid confirmation bias during data interpretation .
Q. Methodological Best Practices
- Experimental design : Include triplicate biological replicates and randomized treatment groups to account for variability .
- Data reporting : Follow FAIR principles (Findable, Accessible, Interoperable, Reusable) by depositing raw sequencing data in public repositories like GEO .
- Ethical compliance : Document RNA source materials (e.g., cell lines, patient-derived samples) in accordance with institutional review board protocols .
Properties
Molecular Formula |
C22H21NO7S |
---|---|
Molecular Weight |
443.5 g/mol |
IUPAC Name |
(2,5-dioxopyrrolidin-1-yl) 3-[(2,5,9-trimethyl-7-oxofuro[3,2-g]chromen-3-yl)methylsulfanyl]propanoate |
InChI |
InChI=1S/C22H21NO7S/c1-11-8-20(27)29-21-12(2)22-15(9-14(11)21)16(13(3)28-22)10-31-7-6-19(26)30-23-17(24)4-5-18(23)25/h8-9H,4-7,10H2,1-3H3 |
InChI Key |
OOLVYUSWNMDHMP-UHFFFAOYSA-N |
Canonical SMILES |
CC1=CC(=O)OC2=C(C3=C(C=C12)C(=C(O3)C)CSCCC(=O)ON4C(=O)CCC4=O)C |
Origin of Product |
United States |
Disclaimer and Information on In-Vitro Research Products
Please be aware that all articles and product information presented on BenchChem are intended solely for informational purposes. The products available for purchase on BenchChem are specifically designed for in-vitro studies, which are conducted outside of living organisms. In-vitro studies, derived from the Latin term "in glass," involve experiments performed in controlled laboratory settings using cells or tissues. It is important to note that these products are not categorized as medicines or drugs, and they have not received approval from the FDA for the prevention, treatment, or cure of any medical condition, ailment, or disease. We must emphasize that any form of bodily introduction of these products into humans or animals is strictly prohibited by law. It is essential to adhere to these guidelines to ensure compliance with legal and ethical standards in research and experimentation.