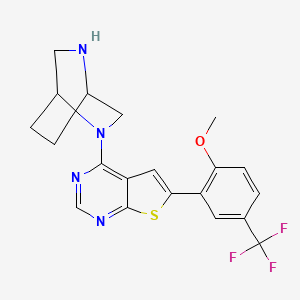
KRAS G12D inhibitor 14
- Click on QUICK INQUIRY to receive a quote from our team of experts.
- With the quality product at a COMPETITIVE price, you can focus more on your research.
Overview
Description
KRAS G12D mutations are among the most prevalent oncogenic drivers in pancreatic (35%), colorectal (13%), and non-small cell lung cancers (4%) . KRAS G12D inhibitor 14 (hypothetically designated here as a representative compound based on evidence) exemplifies a novel class of inhibitors targeting the aspartate residue at position 12. These inhibitors exploit unique mechanisms such as salt bridge formation, covalent binding, or allosteric modulation to disrupt KRAS signaling. For instance, TH-Z835, a bicyclic piperazine derivative, forms a salt bridge with Asp12, inducing a switch-II pocket in KRAS G12D . Similarly, MRTX1133 (non-covalent) and RMC-9805 (covalent tri-complex) bind with high selectivity to inhibit both GDP- and GTP-bound KRAS G12D .
Preparation Methods
Synthetic Routes and Reaction Conditions
The synthesis of KRAS G12D inhibitor 14 involves multiple steps, including the formation of key intermediates and final coupling reactions. Common reaction conditions include the use of organic solvents, catalysts, and temperature control to ensure high yield and purity of the final product .
Industrial Production Methods
Industrial production of this compound involves scaling up the laboratory synthesis to a larger scale while maintaining the quality and consistency of the compound . This process often requires optimization of reaction conditions, purification techniques, and quality control measures to meet regulatory standards .
Chemical Reactions Analysis
Key Structural Modifications Leading to Compound 14
The development of ERAS-5024 involved iterative optimization of the quinazoline scaffold:
-
C6 Position Substitution : Replacement of a chlorine atom in compound 9 with a CF₃ group enhanced hydrophobic interactions within the pocket formed by Thr58, Arg68, and Met72 .
-
Cyano Substituent on the A Ring : Introduced a direct hydrogen bond with the backbone NH of Glu63 .
-
Bridged Amine Tail : Facilitated dual interactions with the Asp12 carboxylate side chain and the Gly60 backbone carbonyl, critical for selectivity over wild-type (WT) KRAS .
Crystallographic Analysis of Binding Interactions
A 2.25 Å resolution crystal structure of compound 14 bound to KRAS G12D (PDB ID: N/A) revealed:
-
Region 1 (Asp12 Interaction) :
-
Region 2 (SWII Pocket) :
-
Region 3 (P-Loop Stabilization) :
Biochemical and Cellular Efficacy
Mechanism of Action
-
GTP-State Targeting : Unlike KRAS G12C inhibitors, ERAS-5024 binds both GDP- and GTP-bound states of KRAS G12D, disrupting effector interactions (e.g., RAF-RBD) .
-
Covalent vs. Noncovalent : ERAS-5024 is noncovalent, while recent covalent inhibitors (e.g., Compound 1 in ) alkylate Asp12, forming stable ester bonds.
Synergistic Combinations
-
Farnesyl-Transferase Inhibitors (FTIs) : Co-treatment with FTIs (e.g., tipifarnib) enhances efficacy in resistant KRAS G12D PDAC models by inhibiting HRAS/RHEB farnesylation .
-
Immune Modulation : ERAS-5024 increases intratumoral M1-like macrophages and CD8⁺ T cells, suggesting combinatorial potential with immunotherapies .
Comparative Analysis with Other KRAS G12D Inhibitors
Inhibitor | Mechanism | Selectivity | Clinical Stage |
---|---|---|---|
ERAS-5024 | Noncovalent S-IIP binding | 188-fold | Preclinical |
MRTX1133 | Noncovalent S-IIP binding | >100-fold | Phase I |
TH-Z835 | Salt bridge with Asp12 | Moderate | Preclinical |
Scientific Research Applications
In Vitro Studies
In vitro experiments have shown that KRAS G12D inhibitor 14 effectively induces apoptosis in cancer cell lines with the G12D mutation. For instance:
- Cell Lines Tested : CT26 and SW1990 exhibited significant reductions in active KRAS levels and downstream signaling pathways when treated with the inhibitor.
- Mechanistic Insights : The compound down-regulates phosphorylated Raf and Erk levels specifically in KRAS G12D mutated cells .
In Vivo Efficacy
In vivo studies demonstrate the antitumor efficacy of this compound in mouse models:
- Tumor Models : The compound was tested in a CT26 tumor model, resulting in tumor growth inhibition (TGI) rates of 42% to 53% without apparent toxicity at doses of 40 mg/kg or 60 mg/kg administered intraperitoneally .
- Long-term Effects : These findings suggest that the compound not only inhibits tumor growth but also has the potential for long-term therapeutic benefits.
Case Studies and Clinical Implications
Recent research highlights the potential for combining this compound with other therapeutic agents to enhance efficacy:
- Combination Therapies : Studies indicate that combining inhibitors targeting different pathways may overcome resistance mechanisms observed in monotherapy settings. For example, integrating immune checkpoint inhibitors could enhance antitumor responses .
- Clinical Trials : The promising results from preclinical models have led to discussions about advancing these inhibitors into clinical trials, particularly for pancreatic ductal adenocarcinoma (PDAC) patients where KRAS mutations are prevalent .
Comparative Data Table
Feature | This compound | Other KRAS Inhibitors |
---|---|---|
Binding Affinity (KD) | 33 nM | Varies |
IC50 (Cancer Cell Lines) | 2.1 μM | Varies |
Tumor Growth Inhibition | 42%-53% | Varies |
Induced Apoptosis | Yes | Yes |
Target Specificity | KRAS G12D only | Varies |
Mechanism of Action
KRAS G12D inhibitor 14 exerts its effects by binding to the KRAS G12D mutant protein and inhibiting its activity . This binding disrupts the interaction between KRAS G12D and its downstream effectors, such as RAF1, thereby blocking the activation of signaling pathways like the mitogen-activated protein kinase (MAPK) pathway . The inhibition of these pathways leads to reduced cancer cell proliferation and increased apoptosis .
Comparison with Similar Compounds
Mechanism of Action and Binding Modes
Key Insights :
- TH-Z835 binds GDP- and GTP-bound KRAS G12D with similar affinity (KD ~4.4 μM) but exhibits off-target effects on Rho/Ran GTPases .
- MRTX1133 achieves >1,000-fold selectivity for G12D over WT KRAS, with single-digit nM potency in vitro .
- RMC-9805 leverages Cyclophilin A to enhance cellular penetration and covalent engagement of Asp12, showing efficacy in pancreatic ductal adenocarcinoma (PDAC) models .
Selectivity and Efficacy
Key Insights :
- MRTX1133 demonstrates superior potency and specificity, with in vivo efficacy in reducing tumor volume by 70–80% .
- TH-Z835 shows moderate anti-proliferative effects but lacks clinical advancement due to off-target activity .
Pharmacokinetics and Clinical Progress
Inhibitor | Bioavailability | Half-Life (h) | Clinical Stage |
---|---|---|---|
MRTX1133 | Low (IV only) | >50 | Preclinical |
RMC-9805 | High (oral) | 24–36 | Phase I (NCT05578092) |
SHR1127 | Oral | 8–12 | Preclinical |
Key Insights :
Biological Activity
KRAS mutations, particularly the G12D variant, are prevalent in various cancers, notably pancreatic ductal adenocarcinoma (PDAC). The development of targeted inhibitors has become a focal point in cancer therapy. This article examines the biological activity of KRAS G12D inhibitor 14 , also known as ACA-14, focusing on its mechanism of action, efficacy in preclinical models, and potential therapeutic implications.
ACA-14 functions primarily by inhibiting nucleotide exchange and disrupting the binding of KRAS to its effectors. It has been shown to:
- Modulate Nucleotide Exchange: ACA-14 decreases both intrinsic and SOS-mediated GDP/GTP exchange reactions in a dose-dependent manner, with effective concentrations (EC50) of approximately 3.8 µM and 2.1 µM, respectively .
- Inhibit Effector Binding: The compound reduces the affinity between KRAS and its effector c-Raf, which is critical for downstream signaling in the MAPK pathway. This inhibition occurs through direct binding to KRAS, targeting specific residues that facilitate effector interaction .
Efficacy in Preclinical Models
The efficacy of ACA-14 has been evaluated across various models:
- Cell Line Studies:
-
Animal Models:
- In xenograft models of PDAC, ACA-14 induced substantial tumor regression and apoptosis in KRAS G12D-mutant tumors. The treatment led to changes in the tumor microenvironment, including alterations in fibroblast and macrophage populations .
- Notably, T-cell involvement was crucial for the full antitumor effect, suggesting that immunomodulation may play a role in its therapeutic efficacy .
Comparative Analysis with Other Inhibitors
The table below summarizes key characteristics of ACA-14 compared to other notable KRAS G12D inhibitors such as MRTX1133:
Compound | Type | Affinity (Kd) | Efficacy | Mechanism |
---|---|---|---|---|
ACA-14 | Noncovalent | ~6 µM | Significant tumor regression | Disrupts effector binding |
MRTX1133 | Noncovalent | ~0.5 µM | Complete remissions in models | Directly inhibits KRAS signaling |
Compound 3 | Noncovalent | Sub-nanomolar | Strong anti-tumor activity | Targets switch II pocket |
Case Studies
Recent studies have highlighted the potential of ACA-14 and similar inhibitors:
- In a study involving PDAC models, ACA-14 was shown to significantly impact tumor growth dynamics when combined with immunotherapeutic agents .
- Another case demonstrated that dual inhibition strategies involving ACA-14 could enhance therapeutic outcomes by targeting both KRAS and associated signaling pathways .
Q & A
Basic Research Questions
Q. What is the mechanism of action of KRAS G12D inhibitor 14, and how does it achieve mutant-specific binding?
this compound binds selectively to the GTP-bound active state of KRAS G12D, stabilizing an inactive conformation by forming a salt bridge interaction with the aspartic acid residue at position 12. This disrupts downstream signaling pathways like MAPK/ERK. Structural studies using X-ray crystallography (PDB: 7EW9) confirmed the interaction between the inhibitor's alkylamine group and the negatively charged carboxylate of G12D . Biochemical assays (e.g., SOS-catalyzed nucleotide exchange) further validated its potency (Kd = 33 nM) and selectivity over KRAS G13D .
Q. Which experimental assays are recommended to evaluate the inhibitory activity of this compound?
Key assays include:
- Nucleotide exchange assays : Monitor GDP/GTP cycling using BODIPY®-GDP (e.g., KRAS(G12D) Nucleotide Exchange Assay Kit) to quantify inhibition of GTP loading .
- Cellular proliferation assays : Use CellTiter-Glo in KRAS G12D-mutant pancreatic (e.g., MIA PaCa-2) or colorectal cancer cell lines to assess dose-dependent growth suppression .
- Western blotting : Measure phospho-ERK levels to confirm downstream pathway inhibition .
Q. What distinguishes this compound from covalent KRAS G12C inhibitors like Sotorasib?
Unlike covalent G12C inhibitors that target a cysteine residue, this compound relies on non-covalent interactions (e.g., salt bridges) due to the absence of a reactive cysteine in G12D. This necessitates distinct structural optimization strategies to enhance binding affinity and residence time .
Advanced Research Questions
Q. How can researchers address discrepancies in preclinical efficacy data between in vitro and in vivo models for this compound?
Discrepancies often arise from differences in drug bioavailability or tumor microenvironment complexity. To mitigate:
- Use pharmacodynamic markers : Assess p-ERK suppression in tumors post-treatment to confirm target engagement .
- Optimize dosing regimens: For example, GFH375 (a related inhibitor) showed tumor regression at 10–30 mg/kg BID in xenograft models, emphasizing the need for pharmacokinetic profiling .
- Validate in orthotopic or patient-derived xenograft (PDX) models, which better mimic human tumor biology .
Q. What structural modifications could improve the potency of this compound?
Cyclization strategies (e.g., TH-Z827 derivatives) enhance binding by rigidifying the inhibitor's conformation, as shown in ITC assays and crystallographic studies. Introducing bicyclic moieties improves ΔG values (e.g., ΔG = -9.2 kcal/mol for TH-Z827) by optimizing entropy-enthalpy balance .
Q. How do researchers reconcile conflicting clinical trial outcomes for KRAS G12D inhibitors (e.g., HRS-4642 vs. INCB161734)?
Discrepancies may stem from variations in drug selectivity or patient stratification. For example:
- HRS-4642 : Limited efficacy in early trials due to poor target engagement or off-target effects .
- INCB161734 : Preclinical data showed strong tissue enrichment in pancreatic and lung tumors, suggesting optimized delivery may improve clinical outcomes . Methodological recommendation: Use companion diagnostics (e.g., fluorescent probe kits) to validate target inhibition in patient samples .
Q. What combination therapies could synergize with this compound?
Preclinical data for GFH375 demonstrated synergy with avutometinib (RAF/MEK clamp), reducing tumor burden in KRAS G12D PDAC models. Mechanistically, dual inhibition of KRAS and downstream effectors prevents pathway reactivation . Other candidates include:
- Immune checkpoint inhibitors : Enhance T-cell infiltration in "cold" KRAS-mutant tumors.
- PARP inhibitors : Exploit synthetic lethality in DNA repair-deficient cancers .
Q. Methodological Resources
Table 1: Key Assays for KRAS G12D Inhibitor Profiling
Table 2: Preclinical Models for Efficacy Testing
Model Type | Utility | Example |
---|---|---|
Subcutaneous CDX | Rapid screening of tumor growth inhibition | MIA PaCa-2 (pancreatic) |
Orthotopic PDX | Mimic metastatic spread and microenvironment | CRC PDX models |
Intracranial | Assess CNS penetration | KRAS G12D glioblastoma models |
Properties
Molecular Formula |
C20H19F3N4OS |
---|---|
Molecular Weight |
420.5 g/mol |
IUPAC Name |
4-(2,5-diazabicyclo[2.2.2]octan-2-yl)-6-[2-methoxy-5-(trifluoromethyl)phenyl]thieno[2,3-d]pyrimidine |
InChI |
InChI=1S/C20H19F3N4OS/c1-28-16-5-2-11(20(21,22)23)6-14(16)17-7-15-18(25-10-26-19(15)29-17)27-9-12-3-4-13(27)8-24-12/h2,5-7,10,12-13,24H,3-4,8-9H2,1H3 |
InChI Key |
DYYANULKLUNJSO-UHFFFAOYSA-N |
Canonical SMILES |
COC1=C(C=C(C=C1)C(F)(F)F)C2=CC3=C(N=CN=C3S2)N4CC5CCC4CN5 |
Origin of Product |
United States |
Disclaimer and Information on In-Vitro Research Products
Please be aware that all articles and product information presented on BenchChem are intended solely for informational purposes. The products available for purchase on BenchChem are specifically designed for in-vitro studies, which are conducted outside of living organisms. In-vitro studies, derived from the Latin term "in glass," involve experiments performed in controlled laboratory settings using cells or tissues. It is important to note that these products are not categorized as medicines or drugs, and they have not received approval from the FDA for the prevention, treatment, or cure of any medical condition, ailment, or disease. We must emphasize that any form of bodily introduction of these products into humans or animals is strictly prohibited by law. It is essential to adhere to these guidelines to ensure compliance with legal and ethical standards in research and experimentation.