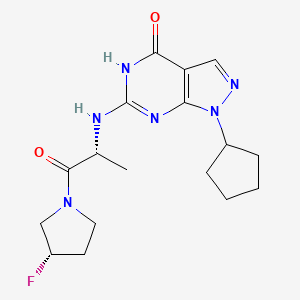
Pde9-IN-1
- Click on QUICK INQUIRY to receive a quote from our team of experts.
- With the quality product at a COMPETITIVE price, you can focus more on your research.
Overview
Description
PDE9-IN-1 is a selective inhibitor of phosphodiesterase 9, an enzyme that specifically hydrolyzes cyclic guanosine monophosphate. This compound has garnered significant attention due to its potential therapeutic applications in various diseases, including neurodegenerative disorders, cardiovascular diseases, and metabolic syndromes .
Preparation Methods
Synthetic Routes and Reaction Conditions
The synthesis of PDE9-IN-1 involves several steps, starting with the preparation of the core structure, followed by functional group modifications to enhance its selectivity and potency. One common synthetic route includes the formation of a pyrazolo[3,4-d]pyrimidine core, which is then functionalized with various substituents to achieve the desired inhibitory activity .
Industrial Production Methods
Industrial production of this compound typically involves large-scale synthesis using optimized reaction conditions to ensure high yield and purity. This includes the use of automated reactors and continuous flow systems to streamline the synthesis process and minimize the production of by-products .
Chemical Reactions Analysis
Types of Reactions
PDE9-IN-1 undergoes several types of chemical reactions, including:
Oxidation: This reaction can modify the functional groups on the compound, potentially altering its inhibitory activity.
Reduction: This reaction can be used to reduce specific functional groups, enhancing the compound’s stability.
Common Reagents and Conditions
Common reagents used in these reactions include oxidizing agents like potassium permanganate, reducing agents like sodium borohydride, and various nucleophiles for substitution reactions. The reactions are typically carried out under controlled conditions, such as specific temperatures and pH levels, to ensure optimal results .
Major Products Formed
The major products formed from these reactions include various derivatives of this compound, each with unique properties and potential therapeutic applications. These derivatives are often tested for their inhibitory activity and selectivity to identify the most promising candidates for further development .
Scientific Research Applications
PDE9-IN-1 has a wide range of scientific research applications, including:
Chemistry: Used as a tool compound to study the role of phosphodiesterase 9 in various biochemical pathways.
Biology: Investigated for its effects on cellular signaling and gene expression.
Medicine: Explored as a potential therapeutic agent for treating neurodegenerative disorders, cardiovascular diseases, and metabolic syndromes.
Industry: Utilized in the development of new drugs and therapeutic strategies.
Mechanism of Action
PDE9-IN-1 exerts its effects by selectively inhibiting phosphodiesterase 9, an enzyme responsible for hydrolyzing cyclic guanosine monophosphate. By inhibiting this enzyme, this compound increases the levels of cyclic guanosine monophosphate within cells, leading to enhanced signaling through the cyclic guanosine monophosphate-dependent pathways. This can result in various physiological effects, such as improved cognitive function, reduced inflammation, and enhanced cardiovascular health .
Comparison with Similar Compounds
Similar Compounds
Some compounds similar to PDE9-IN-1 include:
BAY 73-6691: Another selective inhibitor of phosphodiesterase 9 with similar therapeutic potential.
PF-04447943: A potent inhibitor of phosphodiesterase 9 that has been studied for its effects on cognitive function and neuroprotection.
Uniqueness
This compound is unique due to its high selectivity and potency as a phosphodiesterase 9 inhibitor. Its unique structural features, such as the specific functional groups and core structure, contribute to its superior inhibitory activity and therapeutic potential compared to other similar compounds .
Properties
Molecular Formula |
C17H23FN6O2 |
---|---|
Molecular Weight |
362.4 g/mol |
IUPAC Name |
1-cyclopentyl-6-[[(2R)-1-[(3S)-3-fluoropyrrolidin-1-yl]-1-oxopropan-2-yl]amino]-5H-pyrazolo[3,4-d]pyrimidin-4-one |
InChI |
InChI=1S/C17H23FN6O2/c1-10(16(26)23-7-6-11(18)9-23)20-17-21-14-13(15(25)22-17)8-19-24(14)12-4-2-3-5-12/h8,10-12H,2-7,9H2,1H3,(H2,20,21,22,25)/t10-,11+/m1/s1 |
InChI Key |
HOQGZKUBNCAZBE-MNOVXSKESA-N |
Isomeric SMILES |
C[C@H](C(=O)N1CC[C@@H](C1)F)NC2=NC3=C(C=NN3C4CCCC4)C(=O)N2 |
Canonical SMILES |
CC(C(=O)N1CCC(C1)F)NC2=NC3=C(C=NN3C4CCCC4)C(=O)N2 |
Origin of Product |
United States |
Disclaimer and Information on In-Vitro Research Products
Please be aware that all articles and product information presented on BenchChem are intended solely for informational purposes. The products available for purchase on BenchChem are specifically designed for in-vitro studies, which are conducted outside of living organisms. In-vitro studies, derived from the Latin term "in glass," involve experiments performed in controlled laboratory settings using cells or tissues. It is important to note that these products are not categorized as medicines or drugs, and they have not received approval from the FDA for the prevention, treatment, or cure of any medical condition, ailment, or disease. We must emphasize that any form of bodily introduction of these products into humans or animals is strictly prohibited by law. It is essential to adhere to these guidelines to ensure compliance with legal and ethical standards in research and experimentation.