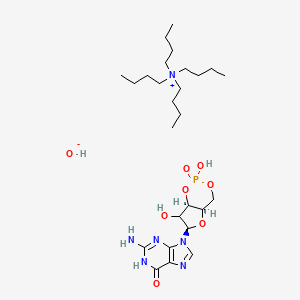
Cyclic GMP (TBAOH)
- Click on QUICK INQUIRY to receive a quote from our team of experts.
- With the quality product at a COMPETITIVE price, you can focus more on your research.
Overview
Description
Cyclic guanosine monophosphate (Tetra-n-butylammonium hydroxide) is a cyclic nucleotide derived from guanosine triphosphate. It acts as a second messenger in various biological processes, including vasodilation, neurotransmission, and apoptosis. Cyclic guanosine monophosphate is known for its role in regulating intracellular protein kinases and ion channels, making it a crucial component in cellular signaling pathways .
Preparation Methods
Synthetic Routes and Reaction Conditions
Cyclic guanosine monophosphate can be synthesized through the catalytic conversion of guanosine triphosphate by guanylate cyclase enzymes. The reaction typically involves the activation of guanylate cyclase by nitric oxide or natriuretic peptides, leading to the formation of cyclic guanosine monophosphate .
Industrial Production Methods
Industrial production of cyclic guanosine monophosphate often involves microbial fermentation processes. For instance, engineered strains of Escherichia coli can be used to overexpress dinucleotide cyclases, which catalyze the formation of cyclic guanosine monophosphate. The product is then purified using techniques such as affinity chromatography and reverse-phase liquid chromatography .
Chemical Reactions Analysis
Types of Reactions
Cyclic guanosine monophosphate undergoes various chemical reactions, including:
Oxidation: Cyclic guanosine monophosphate can be oxidized to form guanosine monophosphate.
Reduction: Reduction reactions can convert cyclic guanosine monophosphate back to guanosine triphosphate.
Substitution: Substitution reactions can occur at the phosphate group, leading to the formation of different cyclic nucleotides.
Common Reagents and Conditions
Oxidation: Hydrogen peroxide or other oxidizing agents.
Reduction: Reducing agents such as sodium borohydride.
Substitution: Various nucleophiles can be used to substitute the phosphate group.
Major Products Formed
Oxidation: Guanosine monophosphate.
Reduction: Guanosine triphosphate.
Substitution: Different cyclic nucleotides depending on the nucleophile used.
Scientific Research Applications
Cyclic guanosine monophosphate has a wide range of scientific research applications:
Chemistry: Used as a model compound to study nucleotide interactions and signaling pathways.
Biology: Plays a crucial role in cellular signaling, regulating processes such as vasodilation, neurotransmission, and apoptosis.
Medicine: Investigated for its potential therapeutic applications in cardiovascular diseases, erectile dysfunction, and certain types of cancer.
Industry: Used in the development of pharmaceuticals and as a research tool in various biochemical assays
Mechanism of Action
Cyclic guanosine monophosphate exerts its effects by activating intracellular protein kinases, such as protein kinase G. This activation leads to the phosphorylation of target proteins, resulting in various cellular responses. Cyclic guanosine monophosphate also regulates ion channels and phosphodiesterases, further influencing cellular processes. The primary molecular targets include guanylate cyclase, protein kinase G, and cyclic nucleotide-gated ion channels .
Comparison with Similar Compounds
Similar Compounds
Cyclic adenosine monophosphate: Another cyclic nucleotide that acts as a second messenger in cellular signaling.
Guanosine monophosphate: A nucleotide that can be converted to cyclic guanosine monophosphate.
Guanosine triphosphate: The precursor molecule for cyclic guanosine monophosphate synthesis.
Uniqueness
Cyclic guanosine monophosphate is unique due to its specific role in regulating protein kinase G and cyclic nucleotide-gated ion channels. Unlike cyclic adenosine monophosphate, which primarily activates protein kinase A, cyclic guanosine monophosphate has distinct molecular targets and pathways, making it a critical component in various physiological processes .
Properties
Molecular Formula |
C26H49N6O8P |
---|---|
Molecular Weight |
604.7 g/mol |
IUPAC Name |
9-[(4aR,6R,7aR)-2,7-dihydroxy-2-oxo-4a,6,7,7a-tetrahydro-4H-furo[3,2-d][1,3,2]dioxaphosphinin-6-yl]-2-amino-1H-purin-6-one;tetrabutylazanium;hydroxide |
InChI |
InChI=1S/C16H36N.C10H12N5O7P.H2O/c1-5-9-13-17(14-10-6-2,15-11-7-3)16-12-8-4;11-10-13-7-4(8(17)14-10)12-2-15(7)9-5(16)6-3(21-9)1-20-23(18,19)22-6;/h5-16H2,1-4H3;2-3,5-6,9,16H,1H2,(H,18,19)(H3,11,13,14,17);1H2/q+1;;/p-1/t;3-,5?,6+,9-;/m.1./s1 |
InChI Key |
MLRIRCVSOJNWQD-LOAXFSTJSA-M |
Isomeric SMILES |
CCCC[N+](CCCC)(CCCC)CCCC.C1[C@@H]2[C@@H](C([C@@H](O2)N3C=NC4=C3N=C(NC4=O)N)O)OP(=O)(O1)O.[OH-] |
Canonical SMILES |
CCCC[N+](CCCC)(CCCC)CCCC.C1C2C(C(C(O2)N3C=NC4=C3N=C(NC4=O)N)O)OP(=O)(O1)O.[OH-] |
Origin of Product |
United States |
Disclaimer and Information on In-Vitro Research Products
Please be aware that all articles and product information presented on BenchChem are intended solely for informational purposes. The products available for purchase on BenchChem are specifically designed for in-vitro studies, which are conducted outside of living organisms. In-vitro studies, derived from the Latin term "in glass," involve experiments performed in controlled laboratory settings using cells or tissues. It is important to note that these products are not categorized as medicines or drugs, and they have not received approval from the FDA for the prevention, treatment, or cure of any medical condition, ailment, or disease. We must emphasize that any form of bodily introduction of these products into humans or animals is strictly prohibited by law. It is essential to adhere to these guidelines to ensure compliance with legal and ethical standards in research and experimentation.