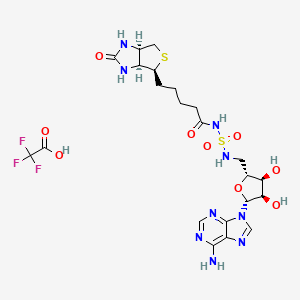
Bio-ams tfa
- Click on QUICK INQUIRY to receive a quote from our team of experts.
- With the quality product at a COMPETITIVE price, you can focus more on your research.
Overview
Description
Bio-AMS (TFA) is a potent inhibitor of bacterial biotin protein ligase. It exhibits selective activity against Mycobacterium tuberculosis and disrupts fatty acid and lipid biosynthesis . This compound is primarily used in research settings to study its effects on bacterial growth and metabolism.
Preparation Methods
Synthetic Routes and Reaction Conditions
Bio-AMS (TFA) is synthesized through a series of chemical reactions involving the use of trifluoroacetic acid. The synthesis typically involves the following steps:
Acidification: The initial step involves acidification with pure trifluoroacetic acid.
Neutralization: This is followed by neutralization using 2M TrisBase.
Digestion: The final step involves digestion to obtain the desired compound.
Industrial Production Methods
The industrial production of Bio-AMS (TFA) follows similar steps but on a larger scale. The process is optimized to ensure high yield and purity of the compound. The use of trifluoroacetic acid in the production process ensures efficient extraction and purification of the compound .
Chemical Reactions Analysis
Types of Reactions
Bio-AMS (TFA) undergoes several types of chemical reactions, including:
Oxidation: This reaction involves the addition of oxygen to the compound, leading to the formation of oxidized products.
Reduction: This reaction involves the removal of oxygen or the addition of hydrogen, resulting in reduced products.
Substitution: This reaction involves the replacement of one functional group with another, leading to the formation of substituted products.
Common Reagents and Conditions
The common reagents used in these reactions include oxidizing agents like hydrogen peroxide, reducing agents like sodium borohydride, and various substituting agents. The reactions are typically carried out under controlled conditions to ensure the desired products are formed .
Major Products Formed
The major products formed from these reactions include oxidized, reduced, and substituted derivatives of Bio-AMS (TFA). These products are often used in further research to study their effects on bacterial growth and metabolism .
Scientific Research Applications
Bio-AMS (TFA) has several scientific research applications, including:
Chemistry: It is used to study the effects of biotin protein ligase inhibition on bacterial growth and metabolism.
Biology: It is used to investigate the role of biotin protein ligase in various biological processes.
Medicine: It is used in the development of new antibiotics targeting Mycobacterium tuberculosis.
Industry: It is used in the production of various biotechnological products.
Mechanism of Action
Bio-AMS (TFA) exerts its effects by inhibiting bacterial biotin protein ligase. This inhibition disrupts fatty acid and lipid biosynthesis, leading to the arrest of bacterial growth. The molecular targets involved in this process include the biotin protein ligase enzyme and various pathways related to fatty acid and lipid biosynthesis .
Comparison with Similar Compounds
Similar Compounds
Some similar compounds to Bio-AMS (TFA) include:
Biotin: A vitamin that acts as a coenzyme in various metabolic processes.
Biotinylated Compounds: Compounds that have been chemically linked to biotin for various research purposes.
Uniqueness
Bio-AMS (TFA) is unique in its selective inhibition of bacterial biotin protein ligase. This selective activity makes it a valuable tool in the study of bacterial growth and metabolism, as well as in the development of new antibiotics targeting Mycobacterium tuberculosis .
properties
Molecular Formula |
C22H30F3N9O9S2 |
---|---|
Molecular Weight |
685.7 g/mol |
IUPAC Name |
5-[(3aS,4S,6aR)-2-oxo-1,3,3a,4,6,6a-hexahydrothieno[3,4-d]imidazol-4-yl]-N-[[(2R,3S,4R,5R)-5-(6-aminopurin-9-yl)-3,4-dihydroxyoxolan-2-yl]methylsulfamoyl]pentanamide;2,2,2-trifluoroacetic acid |
InChI |
InChI=1S/C20H29N9O7S2.C2HF3O2/c21-17-14-18(23-7-22-17)29(8-24-14)19-16(32)15(31)10(36-19)5-25-38(34,35)28-12(30)4-2-1-3-11-13-9(6-37-11)26-20(33)27-13;3-2(4,5)1(6)7/h7-11,13,15-16,19,25,31-32H,1-6H2,(H,28,30)(H2,21,22,23)(H2,26,27,33);(H,6,7)/t9-,10+,11-,13-,15+,16+,19+;/m0./s1 |
InChI Key |
VXWOGYSNAHPLOO-IWTWRERHSA-N |
Isomeric SMILES |
C1[C@H]2[C@@H]([C@@H](S1)CCCCC(=O)NS(=O)(=O)NC[C@@H]3[C@H]([C@H]([C@@H](O3)N4C=NC5=C(N=CN=C54)N)O)O)NC(=O)N2.C(=O)(C(F)(F)F)O |
Canonical SMILES |
C1C2C(C(S1)CCCCC(=O)NS(=O)(=O)NCC3C(C(C(O3)N4C=NC5=C(N=CN=C54)N)O)O)NC(=O)N2.C(=O)(C(F)(F)F)O |
Origin of Product |
United States |
Disclaimer and Information on In-Vitro Research Products
Please be aware that all articles and product information presented on BenchChem are intended solely for informational purposes. The products available for purchase on BenchChem are specifically designed for in-vitro studies, which are conducted outside of living organisms. In-vitro studies, derived from the Latin term "in glass," involve experiments performed in controlled laboratory settings using cells or tissues. It is important to note that these products are not categorized as medicines or drugs, and they have not received approval from the FDA for the prevention, treatment, or cure of any medical condition, ailment, or disease. We must emphasize that any form of bodily introduction of these products into humans or animals is strictly prohibited by law. It is essential to adhere to these guidelines to ensure compliance with legal and ethical standards in research and experimentation.