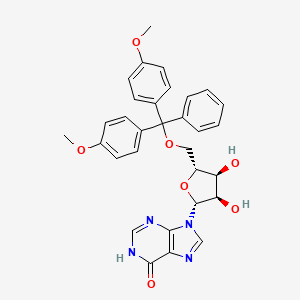
5'-O-DMT-rI
- Click on QUICK INQUIRY to receive a quote from our team of experts.
- With the quality product at a COMPETITIVE price, you can focus more on your research.
Overview
Description
5’-O-DMT-rI, also known as 5’-O-(4,4’-dimethoxytrityl)ribonucleoside, is a modified nucleoside used primarily in the synthesis of oligoribonucleotides. This compound is characterized by the presence of a dimethoxytrityl (DMT) protecting group at the 5’ hydroxyl position of the ribonucleoside, which is crucial for its role in automated RNA synthesis.
Preparation Methods
Synthetic Routes and Reaction Conditions
The synthesis of 5’-O-DMT-rI typically involves the protection of the 5’ hydroxyl group of a ribonucleoside with a dimethoxytrityl chloride (DMT-Cl) reagent. The reaction is usually carried out in the presence of a base such as pyridine or triethylamine to facilitate the formation of the DMT ether linkage. The general reaction scheme is as follows:
Starting Material: Ribonucleoside (e.g., adenosine, cytidine, guanosine, or uridine).
Reagent: Dimethoxytrityl chloride (DMT-Cl).
Base: Pyridine or triethylamine.
Solvent: Dichloromethane (DCM) or acetonitrile.
Reaction Conditions: Room temperature, typically under an inert atmosphere to prevent moisture interference.
The reaction proceeds with the nucleophilic attack of the 5’ hydroxyl group on the DMT-Cl, resulting in the formation of 5’-O-DMT-rI.
Industrial Production Methods
Industrial production of 5’-O-DMT-rI follows similar synthetic routes but on a larger scale. The process involves:
Bulk Handling of Reagents: Large quantities of ribonucleosides and DMT-Cl.
Automated Reaction Systems: Use of automated synthesis equipment to ensure consistent reaction conditions and high yield.
Purification: Typically involves chromatography techniques such as high-performance liquid chromatography (HPLC) to achieve high purity levels required for oligonucleotide synthesis.
Chemical Reactions Analysis
Types of Reactions
5’-O-DMT-rI undergoes several types of chemical reactions, primarily focused on the removal of the DMT protecting group and subsequent modifications to the ribonucleoside:
Deprotection: The DMT group can be removed under acidic conditions, typically using trichloroacetic acid (TCA) in dichloromethane.
Oxidation: The ribonucleoside can undergo oxidation reactions, often using reagents like iodine in the presence of water.
Substitution: The nucleobase can participate in substitution reactions, allowing for further functionalization.
Common Reagents and Conditions
Deprotection: Trichloroacetic acid (TCA) in dichloromethane.
Oxidation: Iodine in water or other oxidizing agents.
Substitution: Various nucleophilic reagents depending on the desired modification.
Major Products
The major products formed from these reactions include the deprotected ribonucleoside and various modified nucleosides depending on the specific substitution reactions performed.
Scientific Research Applications
5’-O-DMT-rI is widely used in scientific research, particularly in the fields of chemistry, biology, and medicine:
Chemistry: Used in the synthesis of oligoribonucleotides, which are essential for studying RNA structure and function.
Biology: Facilitates the creation of RNA sequences for research into gene expression, RNA interference, and ribozyme activity.
Medicine: Plays a role in the development of RNA-based therapeutics, including antisense oligonucleotides and small interfering RNAs (siRNAs).
Industry: Employed in the production of synthetic RNA for various industrial applications, including biotechnology and pharmaceuticals.
Mechanism of Action
The primary mechanism of action for 5’-O-DMT-rI involves its role as a protected intermediate in RNA synthesis. The DMT group protects the 5’ hydroxyl group during the stepwise addition of nucleotides, preventing unwanted side reactions. Once the desired oligoribonucleotide is synthesized, the DMT group is removed under acidic conditions, revealing the free 5’ hydroxyl group necessary for further biological activity.
Comparison with Similar Compounds
Similar Compounds
5’-O-DMT-dA: 5’-O-(4,4’-dimethoxytrityl)deoxyadenosine.
5’-O-DMT-dC: 5’-O-(4,4’-dimethoxytrityl)deoxycytidine.
5’-O-DMT-dG: 5’-O-(4,4’-dimethoxytrityl)deoxyguanosine.
5’-O-DMT-dT: 5’-O-(4,4’-dimethoxytrityl)deoxythymidine.
Uniqueness
5’-O-DMT-rI is unique due to its ribose sugar, which distinguishes it from deoxyribonucleosides. This ribose sugar is crucial for the synthesis of RNA, as opposed to DNA, making 5’-O-DMT-rI specifically valuable for RNA-related research and applications.
Properties
IUPAC Name |
9-[(2R,3R,4S,5R)-5-[[bis(4-methoxyphenyl)-phenylmethoxy]methyl]-3,4-dihydroxyoxolan-2-yl]-1H-purin-6-one |
Source
|
---|---|---|
Source | PubChem | |
URL | https://pubchem.ncbi.nlm.nih.gov | |
Description | Data deposited in or computed by PubChem | |
InChI |
InChI=1S/C31H30N4O7/c1-39-22-12-8-20(9-13-22)31(19-6-4-3-5-7-19,21-10-14-23(40-2)15-11-21)41-16-24-26(36)27(37)30(42-24)35-18-34-25-28(35)32-17-33-29(25)38/h3-15,17-18,24,26-27,30,36-37H,16H2,1-2H3,(H,32,33,38)/t24-,26-,27-,30-/m1/s1 |
Source
|
Source | PubChem | |
URL | https://pubchem.ncbi.nlm.nih.gov | |
Description | Data deposited in or computed by PubChem | |
InChI Key |
PQXVLEMIGAJLIN-BQOYKFDPSA-N |
Source
|
Source | PubChem | |
URL | https://pubchem.ncbi.nlm.nih.gov | |
Description | Data deposited in or computed by PubChem | |
Canonical SMILES |
COC1=CC=C(C=C1)C(C2=CC=CC=C2)(C3=CC=C(C=C3)OC)OCC4C(C(C(O4)N5C=NC6=C5N=CNC6=O)O)O |
Source
|
Source | PubChem | |
URL | https://pubchem.ncbi.nlm.nih.gov | |
Description | Data deposited in or computed by PubChem | |
Isomeric SMILES |
COC1=CC=C(C=C1)C(C2=CC=CC=C2)(C3=CC=C(C=C3)OC)OC[C@@H]4[C@H]([C@H]([C@@H](O4)N5C=NC6=C5N=CNC6=O)O)O |
Source
|
Source | PubChem | |
URL | https://pubchem.ncbi.nlm.nih.gov | |
Description | Data deposited in or computed by PubChem | |
Molecular Formula |
C31H30N4O7 |
Source
|
Source | PubChem | |
URL | https://pubchem.ncbi.nlm.nih.gov | |
Description | Data deposited in or computed by PubChem | |
Molecular Weight |
570.6 g/mol |
Source
|
Source | PubChem | |
URL | https://pubchem.ncbi.nlm.nih.gov | |
Description | Data deposited in or computed by PubChem | |
Disclaimer and Information on In-Vitro Research Products
Please be aware that all articles and product information presented on BenchChem are intended solely for informational purposes. The products available for purchase on BenchChem are specifically designed for in-vitro studies, which are conducted outside of living organisms. In-vitro studies, derived from the Latin term "in glass," involve experiments performed in controlled laboratory settings using cells or tissues. It is important to note that these products are not categorized as medicines or drugs, and they have not received approval from the FDA for the prevention, treatment, or cure of any medical condition, ailment, or disease. We must emphasize that any form of bodily introduction of these products into humans or animals is strictly prohibited by law. It is essential to adhere to these guidelines to ensure compliance with legal and ethical standards in research and experimentation.