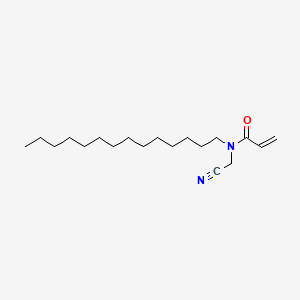
Cyano-myracrylamide
- Click on QUICK INQUIRY to receive a quote from our team of experts.
- With the quality product at a COMPETITIVE price, you can focus more on your research.
Overview
Description
Cyano-myracrylamide (CMA) is a broad-spectrum inhibitor of the Asp-His-His-Cys (DHHC) family of protein acyltransferases, which catalyze protein S-acylation—a lipid post-translational modification critical for membrane trafficking, signaling, and disease progression . Discovered in 2021, CMA was designed to address limitations of earlier DHHC inhibitors, such as 2-bromopalmitate (2BP), by combining potent enzymatic inhibition with reduced cytotoxicity and off-target effects . Its acrylamide warhead enables covalent modification of DHHC enzymes, distinguishing it structurally and mechanistically from lipid-based inhibitors like 2BP .
Preparation Methods
The synthesis of cyano-myracrylamide involves the reaction of tetradecylamine with acrylonitrile under specific conditions . The reaction typically proceeds as follows:
Reactants: Tetradecylamine and acrylonitrile.
Conditions: The reaction is carried out in the presence of a suitable solvent, such as ethanol or dimethylformamide (DMF), at elevated temperatures.
Industrial production methods for this compound are not extensively documented, but the laboratory synthesis provides a foundational approach that can be scaled up with appropriate modifications.
Chemical Reactions Analysis
Cyano-myracrylamide undergoes various chemical reactions, including:
Substitution Reactions: The cyano group can participate in nucleophilic substitution reactions, leading to the formation of amines, carboxylic acids, and ketones.
Radical Reactions: The cyano group acts as a radical acceptor in radical cascade reactions, facilitating the synthesis of complex organic molecules.
Oxidation and Reduction:
Common reagents used in these reactions include strong nucleophiles, radical initiators, and oxidizing or reducing agents. The major products formed depend on the specific reaction conditions and reagents employed.
Scientific Research Applications
Inhibition of Protein S-Acylation
Mechanism of Action:
Cyano-myracrylamide acts by covalently modifying DHHC family proteins, which are responsible for adding lipid groups to proteins through S-acylation. This modification is crucial for various cellular signaling pathways. By inhibiting these enzymes, this compound can disrupt signaling events that are critical for cell function and survival .
Case Studies:
- Cell Signaling Modulation: Research demonstrated that this compound effectively inhibits S-acylation in live cells, leading to altered cellular responses in pathways such as epidermal growth factor receptor (EGFR) signaling and lipid uptake processes mediated by CD36 .
- Therapeutic Implications: The compound has been identified as a potential therapeutic agent against SARS-CoV-2 by inhibiting the S-acylation of viral proteins, thereby reducing infectivity .
Applications in Infectious Disease Research
Targeting Viral Proteins:
this compound has been shown to inhibit the activity of zDHHC enzymes responsible for the palmitoylation of the spike protein of SARS-CoV-2. This inhibition may provide a novel approach to reduce viral infectivity and represents a strategic target in developing antiviral therapies .
Potential for Broader Applications:
The ability of this compound to selectively inhibit specific protein modifications opens avenues for its use in other infectious diseases where similar mechanisms are at play. For instance, targeting palmitoylation pathways may have implications for other viral infections or even bacterial pathogenesis.
Development of Chemical Probes
High-Throughput Screening:
The synthesis of this compound has facilitated the development of high-throughput screening methods aimed at identifying additional inhibitors of DHHC enzymes. This is particularly valuable in drug discovery efforts focused on diseases influenced by protein S-acylation .
Chemical Space Exploration:
Research into variations of this compound continues to explore its chemical space, seeking derivatives that may enhance potency or selectivity against specific targets within the DHHC family .
Safety and Biocompatibility
Toxicity Profile:
Compared to 2-bromopalmitate, this compound exhibits lower toxicity levels while maintaining effective inhibition of target enzymes. This safety profile is crucial for its potential therapeutic applications, especially in clinical settings where patient safety is paramount .
Mechanism of Action
Cyano-myracrylamide exerts its effects by inhibiting the activity of zDHHC20, a member of the DHHC family of protein acyltransferases . The compound binds to the active site of zDHHC20, preventing the enzyme from catalyzing the S-acylation of target proteins . This inhibition disrupts cellular signaling pathways regulated by protein S-acylation, leading to altered cellular functions and potential therapeutic effects .
Comparison with Similar Compounds
Key Competitors in DHHC Inhibition
The primary DHHC inhibitors include:
2-Bromopalmitate (2BP)
Cerulenin
Tunicamycin
Compound V
Cyano-myracrylamide (CMA)
Table 1: Comparative Analysis of DHHC Inhibitors
Mechanistic and Structural Differences
- CMA vs. 2BP: Structure: CMA’s acrylamide group replaces 2BP’s α-halo fatty acid, reducing lipophilicity and non-specific interactions . Selectivity: CMA avoids inhibiting acyl-protein thioesterases (APTs), which reverse S-acylation, whereas 2BP disrupts these "erasers" . Cellular Impact: CMA demonstrates superior live-cell compatibility, enabling prolonged DHHC inhibition without inducing apoptosis at effective doses .
- CMA vs. Cerulenin/Tunicamycin: Cerulenin and tunicamycin target non-DHHC pathways (fatty acid synthase and glycosylation, respectively), making them unsuitable for studying S-acylation-specific roles . CMA’s specificity for DHHCs enhances its utility in mechanistic studies .
In Vitro and In Vivo Efficacy
- Potency : CMA matches 2BP in inhibiting DHHC activity (IC₅₀ ~10–20 µM) but achieves this with 50% lower cytotoxicity in HEK293 and HeLa cells .
- Functional Studies : CMA disrupts DHHC-regulated signaling, such as Wnt/β-catenin pathways, without altering global protein palmitoylation patterns—a limitation of 2BP due to off-target effects .
- Preclinical Data : 2BP has been used in cancer models to validate DHHC inhibition but requires high doses that exacerbate toxicity. CMA’s improved safety profile positions it as a better candidate for therapeutic exploration .
Biological Activity
Cyano-myracrylamide (CMA) is an acrylamide-based compound that has emerged as a significant inhibitor of protein S-acylation, particularly targeting the zinc finger DHHC domain-containing palmitoyltransferases (DHHC-PATs). This article delves into the biological activity of CMA, presenting its mechanism of action, efficacy in various biological contexts, and implications for therapeutic applications.
CMA acts primarily as an inhibitor of the DHHC family of proteins, which are crucial in the post-translational modification known as S-acylation. This modification involves the addition of fatty acid chains to cysteine residues on proteins, influencing their localization and function. CMA's structure includes an acrylamide warhead that allows it to covalently modify DHHC enzymes, thereby inhibiting their activity.
- IC50 Values : CMA exhibits an IC50 value of approximately 1.35 μM against zDHHC20 in cell-free assays, demonstrating its potency compared to traditional inhibitors like 2-bromopalmitate (2BP) .
Efficacy in Live Cells
Research indicates that CMA effectively inhibits S-acylation in live cells. In studies utilizing metabolic labeling with 17-octadecynoic acid, CMA treatment resulted in a dose-dependent decrease in global S-acylation levels . Furthermore, acyl-biotin exchange assays confirmed the global inhibition of S-acylation by CMA.
Table 1: Comparative Efficacy of CMA and 2BP
Compound | Target | IC50 (μM) | Toxicity Level |
---|---|---|---|
This compound (CMA) | zDHHC20 | 1.35 ± 0.26 | Low |
2-Bromopalmitate (2BP) | zDHHC Family | ~6.75 | High |
Cellular Effects and Case Studies
CMA's inhibition of S-acylation has been linked to various cellular processes:
- EGFR Signaling : CMA modulates epidermal growth factor receptor (EGFR)-mediated signaling pathways, potentially impacting cell proliferation and survival .
- Lipid Uptake : Studies have shown that CMA affects CD36-mediated lipid uptake and droplet formation in cells, indicating its role in metabolic regulation .
- Viral Infections : Notably, CMA has been identified as a potential therapeutic agent against SARS-CoV-2 by inhibiting zDHHC9-mediated S-acylation of the viral spike protein, thereby reducing viral infectivity .
Research Findings
Recent studies have highlighted the versatility and effectiveness of CMA across different biological contexts:
- Neuronal Plasticity : In neuronal cultures, CMA has been shown to influence synaptic protein palmitoylation, which is critical for synaptic plasticity and memory formation .
- Inflammatory Responses : Research indicates that CMA can modulate inflammatory responses by inhibiting palmitoylation of gasdermin D, a protein involved in pyroptosis and cytokine release .
Q & A
Basic Research Questions
Q. What established methodologies are recommended for synthesizing Cyano-myracrylamide, and how can reaction conditions be systematically optimized?
- Methodological Answer : Synthesis typically involves acrylamide precursors modified with cyano groups via nucleophilic substitution or radical polymerization. Key steps include:
Precursor Selection : Use acryloyl chloride and cyanide donors (e.g., KCN) under inert atmospheres to avoid side reactions.
Condition Optimization : Vary temperature (40–80°C), solvent polarity (DMF or acetonitrile), and catalyst ratios (e.g., phase-transfer catalysts).
Purity Monitoring : Employ HPLC with UV detection (λ = 220 nm) to track reaction progress .
- Data Table :
Parameter | Range Tested | Optimal Condition | Characterization Technique |
---|---|---|---|
Temperature | 40–80°C | 60°C | NMR (¹H/¹³C) |
Solvent | DMF, MeCN | DMF | FT-IR (C≡N stretch: 2240 cm⁻¹) |
Q. Which analytical techniques are most reliable for characterizing this compound’s structural integrity and purity?
- Methodological Answer :
- Nuclear Magnetic Resonance (NMR) : Confirm cyano group integration (δ 2.8–3.2 ppm for acrylamide protons) and absence of unreacted precursors.
- Fourier-Transform Infrared Spectroscopy (FT-IR) : Identify C≡N stretches (2240–2260 cm⁻¹) and acrylamide backbone (1650–1700 cm⁻¹).
- High-Performance Liquid Chromatography (HPLC) : Assess purity (>95%) using a C18 column with acetonitrile/water gradient .
Q. How should researchers design controlled experiments to evaluate this compound’s stability under varying pH and temperature conditions?
- Methodological Answer :
Variable Isolation : Test pH (4–10) and temperature (25–70°C) independently to avoid confounding factors.
Degradation Metrics : Measure residual monomer via HPLC and track byproducts (e.g., hydrolyzed acrylamide) with LC-MS.
Statistical Validation : Use ANOVA to compare degradation rates across conditions, ensuring p < 0.05 .
Advanced Research Questions
Q. How can contradictions in spectroscopic data (e.g., NMR vs. FT-IR) for this compound derivatives be resolved?
- Methodological Answer :
- Cross-Validation : Replicate experiments under identical conditions and use complementary techniques (e.g., X-ray crystallography for structural confirmation).
- Computational Modeling : Compare experimental spectra with density functional theory (DFT)-predicted vibrational frequencies or chemical shifts .
- Error Analysis : Quantify instrument calibration errors and sample preparation variability .
Q. What strategies integrate computational modeling with experimental data to predict this compound’s reactivity in novel solvents?
- Methodological Answer :
Molecular Dynamics (MD) Simulations : Model solvation effects using software like Gaussian or GROMACS.
Experimental Correlation : Validate predictions via kinetic studies (e.g., rate constants in ionic liquids vs. simulations).
Sensitivity Analysis : Identify solvent parameters (e.g., dielectric constant) most affecting reactivity .
Q. Which statistical approaches minimize Type I errors when screening this compound analogues for bioactivity?
- Methodological Answer :
- False Discovery Rate (FDR) Control : Apply the Benjamini-Hochberg procedure (α = 0.05) to adjust p-values in high-throughput assays .
- Power Analysis : Predefine sample sizes to ensure adequate sensitivity (β < 0.2).
- Reproducibility Checks : Use independent replicates and blinded data analysis .
Q. Methodological Considerations
Properties
Molecular Formula |
C19H34N2O |
---|---|
Molecular Weight |
306.5 g/mol |
IUPAC Name |
N-(cyanomethyl)-N-tetradecylprop-2-enamide |
InChI |
InChI=1S/C19H34N2O/c1-3-5-6-7-8-9-10-11-12-13-14-15-17-21(18-16-20)19(22)4-2/h4H,2-3,5-15,17-18H2,1H3 |
InChI Key |
RIIUZCPEMOKUSJ-UHFFFAOYSA-N |
Canonical SMILES |
CCCCCCCCCCCCCCN(CC#N)C(=O)C=C |
Origin of Product |
United States |
Disclaimer and Information on In-Vitro Research Products
Please be aware that all articles and product information presented on BenchChem are intended solely for informational purposes. The products available for purchase on BenchChem are specifically designed for in-vitro studies, which are conducted outside of living organisms. In-vitro studies, derived from the Latin term "in glass," involve experiments performed in controlled laboratory settings using cells or tissues. It is important to note that these products are not categorized as medicines or drugs, and they have not received approval from the FDA for the prevention, treatment, or cure of any medical condition, ailment, or disease. We must emphasize that any form of bodily introduction of these products into humans or animals is strictly prohibited by law. It is essential to adhere to these guidelines to ensure compliance with legal and ethical standards in research and experimentation.