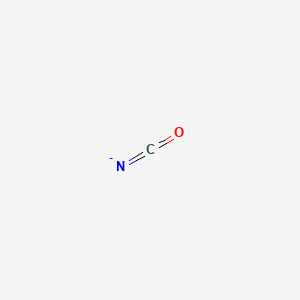
Cyanate
- Click on QUICK INQUIRY to receive a quote from our team of experts.
- With the quality product at a COMPETITIVE price, you can focus more on your research.
Overview
Description
The cyanate ion (OCN⁻) is a linear, resonance-stabilized anion derived from isocyanic acid (HNCO) or cyanic acid (HOCN). It exhibits ambidentate ligand behavior, coordinating to metals via oxygen or nitrogen . Industrially, this compound salts (e.g., NaOCN) are synthesized by heating sodium carbonate with urea . This compound plays a critical role in nitrogen cycling in soils, with concentrations ranging from undetectable to 45 nM in marine environments . Its stability is pH-dependent, favoring persistence in alkaline conditions, while decomposing to ammonium under acidic conditions (pH < 4) .
Preparation Methods
Cyanate can be prepared industrially by heating a mixture of sodium carbonate and urea. The reaction is as follows:
Na2CO3+2OC(NH2)2→2NaNCO+CO2+2NH3+H2O
A similar reaction is used to make potassium this compound . Cyanates are also produced when cyanides are oxidized, a process utilized in cyanide decontamination where oxidants like permanganate and hydrogen peroxide convert toxic cyanide into less-toxic this compound .
Chemical Reactions Analysis
Cyanate undergoes various chemical reactions, including:
Hydrolysis: this compound reacts with water to form ammonia and carbon dioxide.
this compound reacts with amines to form unsymmetrical ureas:Condensation with Amines: HOCN+RNH2→RNHC(O)NH2
Oxidation: Cyanates can be formed by the oxidation of cyanides .
Scientific Research Applications
Scientific Research Applications
1.1 Marine Biogeochemistry
Cyanate serves as a nitrogen source for marine microorganisms, playing a crucial role in the nitrogen cycle. Studies have shown that certain strains of Prochlorococcus and Synechococcus can utilize this compound as their sole nitrogen source, which significantly impacts primary productivity in oceanic environments. For instance, research indicates that Synechococcus dominates cyanase transcripts in non-polar oceans, highlighting the importance of this compound in marine microbial ecology .
1.2 Toxicological Studies
This compound has been identified as a uremic toxin that adversely affects patients with chronic kidney disease. A study developed a liquid chromatography-tandem mass spectrometry (LC-MS-MS) method to quantify this compound levels in rat plasma, providing insights into its systemic exposure and potential toxicity . Additionally, this compound's role in the metabolism of cyanide has been explored, particularly regarding its conversion under sulfur amino acid deficiency, which may contribute to neurodegenerative diseases .
Medical Applications
2.1 Treatment for Sickle Cell Disease
This compound has shown promise in clinical applications for treating sickle cell disease. It reacts with hemoglobin to induce carbamylation, which diminishes the sickling phenomenon of red blood cells. In vitro studies suggest that this process can reduce the percentage of sickled erythrocytes and alleviate painful crises associated with the disease .
2.2 Antiviral Properties
Recent investigations have indicated that potassium this compound exhibits antiviral activity against HIV and herpes viruses by inhibiting viral replication. However, its use in medicine remains controversial due to potential toxicity and side effects .
Agricultural Uses
This compound is utilized as a fertilizer that enhances crop growth and yield. Studies have demonstrated its effectiveness in increasing the growth rates of crops such as potatoes, corn, and wheat . The compound's ability to provide nitrogen makes it valuable for agricultural practices.
Environmental Science
4.1 Soil Microbiology
This compound is actively cycled in soils and serves as a continuous nitrogen source for soil microbes. Research has shown that this compound can be produced from urea amendments in soil, contributing to nitrogen availability for microbial communities .
4.2 Pollution and Remediation
In industrial contexts, this compound is monitored as a pollutant due to its presence alongside other cyanide compounds during processes like mining and electroplating . Understanding this compound's behavior in environmental systems is crucial for developing remediation strategies.
Summary Table of this compound Applications
Case Studies
-
Case Study 1: Marine Microbial Utilization of this compound
A study conducted on non-polar ocean waters revealed that Synechococcus strains dominate cyanase transcripts when utilizing this compound as a nitrogen source. This finding underscores the importance of this compound in marine biogeochemistry and suggests potential implications for oceanic nutrient cycling. -
Case Study 2: Clinical Trials on Sickle Cell Disease
Clinical trials exploring the use of potassium this compound in patients with sickle cell disease demonstrated a reduction in sickling rates among treated individuals. These results support further investigation into the therapeutic potential of this compound derivatives.
Mechanism of Action
Cyanate exerts its effects by binding to the iron atom in cytochrome C oxidase in the mitochondria of cells. This binding prevents cytochrome C oxidase from transporting electrons to oxygen in the electron transport chain of aerobic cellular respiration, effectively inhibiting the production of adenosine triphosphate (ATP) and leading to cell death .
Comparison with Similar Compounds
Comparison with Structurally Related Compounds
Cyanate vs. Urea
- Structure & Reactivity : this compound (OCN⁻) and urea (CO(NH₂)₂) are interconvertible via equilibrium (OCN⁻ + NH₄⁺ ⇌ CO(NH₂)₂), which strongly favors urea (>99%) . Urea isomerization contributes to this compound production in soils, albeit at low rates (~26 pmol g⁻¹ d⁻¹) .
- Environmental Role : this compound is actively cycled in soils, with gross production rates 3–4 orders of magnitude lower than nitrogen mineralization . Urea, in contrast, is a major nitrogen fertilizer but hydrolyzes rapidly via urease.
- Stability : this compound decomposes abiotically to ammonium at pH < 4, while urea remains stable unless enzymatically degraded .
This compound vs. Isocyanates and Thiothis compound
- Structural Isomerism : this compound (-OCN) and isothis compound (-NCO) are functional group isomers. Isocyanates (e.g., methyl isothis compound) are highly reactive, forming polyurethanes, while this compound esters (e.g., phenyl this compound) polymerize into triazines .
- Thiothis compound (SCN⁻) : Thiothis compound shares ambidentate ligand properties but coordinates via sulfur or nitrogen. Uranium/thorium complexes show distinct IR stretches (this compound: ~2199 cm⁻¹; thiothis compound: ~2021–2018 cm⁻¹) . Thiothis compound is less prone to carbamylation reactions compared to this compound .
This compound vs. Fulminate and Cyanide
- Fulminate (CNO⁻): A less stable isomer of this compound, fulminate is explosive due to its metastable structure .
- Cyanide (CN⁻) : Cyanide is far more toxic but can be detoxified via oxidation to this compound, which is ~1000x less toxic .
This compound Esters vs. Other Polymers
This compound ester resins (e.g., PRIMASET®) exhibit superior thermal and mechanical properties compared to epoxy and bismaleimide (BMI) resins:
Property | This compound Ester Composites | BMI Composites | Epoxy Composites |
---|---|---|---|
Compressive Strength* | 236–276 MPa | 193–207 MPa | 193–221 MPa |
Dielectric Constant | 2.6–3.2 | 3.2–4.5 | 4.0–5.0 |
Glass Transition Temp. | 250–290°C | 200–260°C | 120–180°C |
This compound esters are preferred in aerospace and electronics for their low dielectric loss, flame retardancy, and dimensional stability .
Biological Activity
Cyanate, a low-abundance nitrogen compound, has garnered attention due to its significant biological activity across various organisms. This article explores the biological implications of this compound, focusing on its reactivity, enzymatic interactions, and potential applications in bioremediation and microbial metabolism.
This compound (NCO⁻) is known to react with sulfhydryl (–SH) groups in proteins, leading to carbamoylation, which alters the structure and function of proteins. This reactivity is particularly significant in biological systems where enzymes containing –SH groups play crucial roles in metabolic processes. For instance, this compound has been shown to inhibit the activity of sulfurtransferases and other enzymes involved in cysteine metabolism and detoxification pathways .
Enzymatic Interactions
This compound's interaction with enzymes is profound. Key findings include:
- Inhibition of Enzyme Activity : this compound significantly reduces the activity of glutathione peroxidase (GPx) and catalase in rat brain structures. In studies, GPx activity decreased by 70% in the cortex, while catalase activity was reduced by 43% in the cortex and 20% in the striatum upon exposure to this compound .
- Impact on Glutathione Levels : The presence of this compound leads to a decrease in glutathione (GSH) levels, which is critical for cellular antioxidant defense. This reduction is accompanied by an increase in reactive oxygen species (ROS), indicating a prooxidative effect .
Microbial Metabolism of this compound
This compound serves as both a nitrogen and energy source for various microorganisms. Notably:
- Cyanase Enzyme : Many bacteria possess the enzyme cyanase (EC 4.2.1.104), which converts this compound into carbon dioxide and ammonia, facilitating its utilization as a nitrogen source . This process is essential for organisms such as Synechococcus and Prochlorococcus, which are prevalent in marine environments.
- Bioremediation Potential : Certain bacteria like Pseudomonas pseudoalcaligenes can detoxify cyanide through metabolic pathways that involve this compound . These pathways link cyanide degradation with respiratory processes, showcasing the potential for bioremediation applications.
Case Studies
Several studies highlight the biological effects of this compound:
- Neurotoxicity : Research demonstrated that exposure to this compound leads to significant neurotoxic effects, including decreased enzyme activities linked to oxidative stress in rat brain tissues .
- Microbial Growth : In controlled experiments, specific nitrifying bacteria were shown to utilize this compound effectively as an energy source, indicating its role in microbial growth dynamics .
Data Summary
The following table summarizes key findings related to the biological activity of this compound:
Q & A
Q. How can researchers design experiments to investigate cyanate degradation under varying environmental conditions?
Basic Research Focus
To study this compound degradation, a central composite design (CCD) via Response Surface Methodology (RSM) is recommended. This approach evaluates interactions between variables (e.g., pH, temperature, alcohol concentration, storage duration) at multiple levels. For example, experiments can be structured using five levels (−2, −1, 0, +1, +2) for each factor, with responses like this compound concentration measured via HPLC or spectrophotometry. Statistical validation (e.g., ANOVA with F-values, R² > 0.90, and adequate precision > 4) ensures model reliability .
Q. What analytical techniques are most effective for quantifying this compound in complex matrices such as biological or environmental samples?
Basic Research Focus
High-performance liquid chromatography (HPLC) coupled with UV-Vis detection is widely used for this compound quantification due to its sensitivity in aqueous and ethanolic matrices. For kinetic studies, derivatization methods (e.g., reaction with ethanol to form ethyl carbamate) paired with gas chromatography-mass spectrometry (GC-MS) provide precise measurements. Ensure calibration curves are validated against certified standards, and account for matrix effects via spike-recovery tests .
Q. How can computational methods like density functional theory (DFT) model the reaction kinetics of this compound conversion to byproducts such as ethyl carbamate?
Advanced Research Focus
DFT calculations can simulate the thermodynamic and kinetic parameters of this compound reactions. For instance, modeling the hydrolysis of this compound to isocyanic acid (HNCO) under varying pH and temperature conditions reveals activation energies (ΔG‡ ≈ 23.0 kcal/mol) and rate constants (e.g., 8.9 × 10⁻⁵ s⁻¹ at 25°C). Validate computational results against experimental kinetic data (e.g., Arrhenius plots) to refine transition-state geometries .
Q. How should researchers address discrepancies in reported this compound stability across studies, particularly under different storage conditions?
Advanced Research Focus
Discrepancies often arise from uncontrolled variables like trace metal ions or microbial activity. To resolve contradictions:
- Conduct multivariate analysis (e.g., PCA or PLS regression) to identify dominant degradation drivers.
- Replicate experiments under inert atmospheres (e.g., N₂-purged vials) to isolate chemical vs. biological pathways.
- Compare degradation rates using standardized protocols (e.g., ISO 16638-1) and report CV (coefficient of variation) values to assess reproducibility .
Q. What are the key challenges in synthesizing this compound ester resins with tailored thermal and mechanical properties for aerospace applications?
Advanced Research Focus
Challenges include achieving homogeneous dispersion of nanofillers (e.g., carbon nanotubes) in this compound ester matrices and optimizing curing cycles to minimize void formation. Advanced characterization techniques like dynamic mechanical analysis (DMA) and thermogravimetric analysis (TGA) are critical. Radiation resistance studies (e.g., under cosmic radiation) show this compound esters exhibit low erosion rates (<1% mass loss over 5 years), making them suitable for space applications .
Q. What statistical approaches are recommended to analyze this compound’s role in ethyl carbamate (EC) formation during fermentation processes?
Basic Research Focus
Use response surface quadratic models to correlate this compound levels with EC formation. For example, a study with R² = 0.91 and adequate precision = 10.37 identified alcohol concentration and pH as critical factors. Cross-validate models using leave-one-out (LOO) techniques and report prediction intervals to quantify uncertainty .
Q. How do thermodynamic parameters (ΔH, ΔS) influence this compound reactivity in aqueous solutions?
Advanced Research Focus
this compound hydrolysis is entropy-driven (ΔS‡ ≈ −12.1 cal/K·mol) due to solvent reorganization during the transition state. Isothermal titration calorimetry (ITC) can measure enthalpy changes (ΔH‡ ≈ 19.4 kcal/mol), while Eyring plots correlate temperature-dependent rate constants. Note that reactivity decreases with rising pH due to reduced HNCO availability .
Q. What methodologies optimize this compound detection in biological systems with high background interference?
Advanced Research Focus
Pre-concentration via solid-phase extraction (SPE) using anion-exchange cartridges improves detection limits. For in vivo studies, isotope dilution mass spectrometry (ID-MS) with ¹³C-labeled this compound internal standards enhances accuracy. Validate methods using spiked biological fluids (e.g., plasma, urine) and report recovery rates (85–115%) .
Q. How can researchers reconcile contradictory findings on this compound’s environmental persistence in soil vs. aquatic systems?
Advanced Research Focus
Soil studies often underestimate this compound mobility due to adsorption onto clays, while aqueous systems show rapid hydrolysis. Conduct comparative microcosm experiments with controlled redox conditions (e.g., aerobic vs. anaerobic) and quantify this compound speciation (free vs. complexed) using ion chromatography. Meta-analyses of half-life data (t₁/₂) across studies can identify system-specific degradation pathways .
Q. What advanced techniques characterize the interfacial properties of this compound ester composites for high-performance applications?
Advanced Research Focus
Atomic force microscopy (AFM) in tapping mode evaluates nanoscale filler dispersion, while X-ray photoelectron spectroscopy (XPS) assesses surface chemistry post-curing. For radiation resistance, simulate low-Earth orbit conditions using proton irradiation (50–200 keV) and measure erosion yields via quartz crystal microbalance (QCM). Data should be benchmarked against epoxy composites to highlight this compound esters’ superior stability .
Properties
CAS No. |
71000-82-3 |
---|---|
Molecular Formula |
CNO- |
Molecular Weight |
42.017 g/mol |
IUPAC Name |
isocyanate |
InChI |
InChI=1S/CNO/c2-1-3/q-1 |
InChI Key |
IQPQWNKOIGAROB-UHFFFAOYSA-N |
Canonical SMILES |
C(=[N-])=O |
Origin of Product |
United States |
Disclaimer and Information on In-Vitro Research Products
Please be aware that all articles and product information presented on BenchChem are intended solely for informational purposes. The products available for purchase on BenchChem are specifically designed for in-vitro studies, which are conducted outside of living organisms. In-vitro studies, derived from the Latin term "in glass," involve experiments performed in controlled laboratory settings using cells or tissues. It is important to note that these products are not categorized as medicines or drugs, and they have not received approval from the FDA for the prevention, treatment, or cure of any medical condition, ailment, or disease. We must emphasize that any form of bodily introduction of these products into humans or animals is strictly prohibited by law. It is essential to adhere to these guidelines to ensure compliance with legal and ethical standards in research and experimentation.