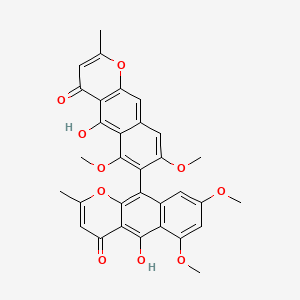
Aurasperone A
- Click on QUICK INQUIRY to receive a quote from our team of experts.
- With the quality product at a COMPETITIVE price, you can focus more on your research.
Overview
Description
Preparation Methods
Synthetic Routes and Reaction Conditions: Aurasperone A can be synthesized through the oxidative coupling of two molecules of naphthopyranone derivatives. The reaction typically involves the use of oxidizing agents such as potassium ferricyanide under controlled conditions to facilitate the dimerization process .
Industrial Production Methods: Industrial production of this compound involves the fermentation of Aspergillus niger strains under optimized conditions to maximize yield. The compound is then extracted and purified using chromatographic techniques .
Types of Reactions:
Oxidation: this compound can undergo oxidation reactions, leading to the formation of various oxidized derivatives.
Reduction: The compound can be reduced to yield different reduced forms, which may exhibit distinct biological activities.
Common Reagents and Conditions:
Oxidizing Agents: Potassium ferricyanide, hydrogen peroxide.
Reducing Agents: Sodium borohydride, lithium aluminum hydride.
Substitution Reagents: Halogens, alkylating agents.
Major Products:
Oxidized Derivatives: Various oxidized forms of this compound.
Reduced Forms: Different reduced derivatives with potential biological activities.
Substituted Compounds: this compound derivatives with substituted functional groups.
Scientific Research Applications
Chemistry: Used as a model compound for studying oxidative coupling reactions and dimerization processes.
Biology: Investigated for its antimicrobial and antiviral properties, particularly against SARS-CoV-2.
Medicine: Explored for its potential therapeutic applications due to its antiviral and antimicrobial activities.
Industry: Potential use in the development of antiviral drugs and antimicrobial agents.
Mechanism of Action
Aurasperone A exerts its antiviral effects primarily by inhibiting the main protease (Mpro) of the SARS-CoV-2 virus. Molecular docking studies have shown that this compound binds to the active site of Mpro, thereby preventing the replication and transcription of the virus . This mechanism is similar to that of other antiviral drugs, making this compound a promising candidate for further development .
Comparison with Similar Compounds
Rubasperone B: Another naphthopyranone derivative with similar antiviral properties.
Fonsecin B: A benzophenone compound with antimicrobial activities.
Dianhydroaurasperone C: A related dimeric naphthopyranone with potential biological activities.
Uniqueness: Aurasperone A stands out due to its potent antiviral activity against SARS-CoV-2, with an inhibitory concentration (IC50) comparable to that of the antiviral drug remdesivir . Additionally, this compound exhibits minimal cytotoxicity, making it a safer alternative for therapeutic applications .
Biological Activity
Aurasperone A, a compound derived from the marine fungus Aspergillus niger, has garnered attention for its significant biological activities, particularly its antiviral properties against SARS-CoV-2. This article synthesizes current research findings regarding the biological activity of this compound, highlighting its mechanisms of action, efficacy, and potential therapeutic applications.
Overview of this compound
This compound belongs to the naphtho-gamma-pyrone class of compounds. Its structure and biological properties have been extensively studied, revealing promising results in various assays.
Antiviral Activity Against SARS-CoV-2
Recent studies have demonstrated that this compound exhibits potent antiviral activity against SARS-CoV-2. The compound was tested in vitro and showed an IC50 of 12.25 µM , which is comparable to the antiviral drug remdesivir (IC50 = 10.11 µM) . The selectivity index (SI), a measure of the safety profile, was calculated at 2641.5 , indicating that this compound is significantly less cytotoxic than remdesivir (SI = 41.07) .
Table 1: Antiviral Activity Comparison
Compound | IC50 (µM) | CC50 (µM) | Selectivity Index |
---|---|---|---|
This compound | 12.25 | 32.36 | 2641.5 |
Remdesivir | 10.11 | 415.22 | 41.07 |
The mechanism by which this compound inhibits SARS-CoV-2 involves targeting the viral main protease (Mpro). Molecular docking studies revealed high binding affinities between this compound and Mpro, with docking scores ranging from −7.0 to −8.1 kcal/mol . The compound forms hydrogen bonds with critical amino acid residues essential for Mpro's catalytic activity, such as Cys-145 and others .
Molecular Dynamics Simulation
To further elucidate the interactions between this compound and Mpro, molecular dynamics simulations were conducted over a period of 150 ns . The results indicated that the compound maintained stable interactions within the active site of Mpro throughout the simulation, confirming its potential as a therapeutic agent against COVID-19 .
Additional Biological Activities
Beyond its antiviral properties, preliminary studies suggest that other members of the aurasperone family may exhibit anti-inflammatory effects. For instance, related compounds have shown dose-dependent inhibition of interleukin-4 (IL-4) signaling pathways, which could have implications for treating inflammatory diseases .
Case Studies and Research Findings
Several research studies have focused on the biological activity of this compound:
- In Vitro Efficacy : In vitro assays demonstrated the compound's ability to inhibit viral replication effectively while exhibiting minimal cytotoxicity.
- Molecular Docking Studies : These studies highlighted the binding interactions with viral proteins, particularly Mpro.
- Comparative Analysis : The efficacy of this compound was compared with other known antiviral agents, establishing its potential as a promising candidate for drug development.
Properties
CAS No. |
1449583-07-6 |
---|---|
Molecular Formula |
C32H26O10 |
Molecular Weight |
570.5 g/mol |
IUPAC Name |
5-hydroxy-7-(5-hydroxy-6,8-dimethoxy-2-methyl-4-oxobenzo[g]chromen-10-yl)-6,8-dimethoxy-2-methylbenzo[g]chromen-4-one |
InChI |
InChI=1S/C32H26O10/c1-13-7-18(33)26-22(41-13)10-15-9-20(38-4)28(31(40-6)23(15)29(26)35)25-17-11-16(37-3)12-21(39-5)24(17)30(36)27-19(34)8-14(2)42-32(25)27/h7-12,35-36H,1-6H3 |
InChI Key |
QAHRSPAZSGMZMT-UHFFFAOYSA-N |
Canonical SMILES |
CC1=CC(=O)C2=C(O1)C=C3C=C(C(=C(C3=C2O)OC)C4=C5C(=C(C6=C4C=C(C=C6OC)OC)O)C(=O)C=C(O5)C)OC |
melting_point |
207 °C |
physical_description |
Solid |
Origin of Product |
United States |
Disclaimer and Information on In-Vitro Research Products
Please be aware that all articles and product information presented on BenchChem are intended solely for informational purposes. The products available for purchase on BenchChem are specifically designed for in-vitro studies, which are conducted outside of living organisms. In-vitro studies, derived from the Latin term "in glass," involve experiments performed in controlled laboratory settings using cells or tissues. It is important to note that these products are not categorized as medicines or drugs, and they have not received approval from the FDA for the prevention, treatment, or cure of any medical condition, ailment, or disease. We must emphasize that any form of bodily introduction of these products into humans or animals is strictly prohibited by law. It is essential to adhere to these guidelines to ensure compliance with legal and ethical standards in research and experimentation.