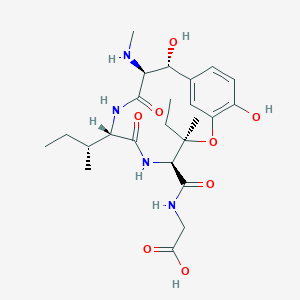
6-Ile-ustiloxin
- Click on QUICK INQUIRY to receive a quote from our team of experts.
- With the quality product at a COMPETITIVE price, you can focus more on your research.
Overview
Description
6-Ile-ustiloxin is a synthetic analog of the ustiloxin family, a group of antimitotic heterodetic cyclopeptides known for their inhibition of tubulin polymerization. Structurally, it features a 13-membered cyclic core with a chiral tertiary alkyl-aryl ether linkage, a hallmark of ustiloxins . The compound is distinguished by the substitution of isoleucine (Ile) at the C-6 position (traditionally occupied by valine [Val] or alanine [Ala] in natural ustiloxins D and F, respectively) . This modification was designed to probe the structure-activity relationship (SAR) of the Val/Ala site, which prior studies suggested influences tubulin-binding efficacy .
Synthetic routes for this compound involve ethynyl aziridine ring-opening reactions and macrolactamization strategies, enabling concise and scalable production . Biological evaluations demonstrate that while the compound retains partial antimitotic activity, its potency is reduced compared to natural ustiloxins, underscoring the sensitivity of the Val/Ala site to structural perturbations .
Preparation Methods
Synthetic Routes and Reaction Conditions: The synthesis of 6-Ile-ustiloxin involves complex organic reactions. One of the methods includes the use of macroporous resin column chromatography and high-speed countercurrent chromatography (HSCCC) for the separation and purification of ustiloxins from rice false smut balls . The process involves extracting ustiloxins using a 3.81% formic acid solution, followed by adsorption on XAD-4 resin. The ustiloxins are then eluted using a 40% methanol solution supplemented with 0.1% trifluoroacetic acid (TFA). Further purification is achieved using HSCCC with a two-phase solvent system consisting of n-butanol, TFA, and water .
Industrial Production Methods: For large-scale production, the adsorption and desorption on macroporous resins such as SP207 and SP700 are effective strategies. The optimal conditions for adsorption include a processing volume of 32 bed volumes (BV), a pH value of 4, and a flow rate of 2 BV/h. For desorption, a 40:60 (v/v) ratio of ethanol to water is used as the eluent, with a volume of 4 BV, a pH value of 4, and a flow rate of 3 BV/h .
Chemical Reactions Analysis
Types of Reactions: 6-Ile-ustiloxin undergoes various chemical reactions, including oxidation, reduction, and substitution
Common Reagents and Conditions: Common reagents used in the reactions involving this compound include oxidizing agents like hydrogen peroxide and reducing agents like sodium borohydride. Substitution reactions often involve nucleophiles such as amines and thiols under mild conditions.
Major Products Formed: The major products formed from these reactions include various derivatives of this compound with modified functional groups. These derivatives are studied for their enhanced biological activities and potential therapeutic applications .
Scientific Research Applications
Chemical Structure and Synthesis
6-Ile-Ustiloxin is part of the ustiloxin family, which includes several cyclic peptides known for their antimitotic properties. The compound's structure features a cyclic tetrapeptide backbone with specific amino acid residues that contribute to its biological activity.
This compound exhibits significant biological activities, primarily as an inhibitor of tubulin polymerization. This property is crucial for its potential use in cancer therapy, as disrupting microtubule dynamics can lead to cell cycle arrest and apoptosis in cancer cells.
Antitumor Activity
Research has demonstrated that ustiloxins, including this compound, can inhibit the growth of various cancer cell lines. For example, studies have reported IC50 values indicating potent cytotoxic effects against lung cancer cells (A-549) and breast adenocarcinoma cells (MCF-7) with values as low as 4.56 µM .
Case Studies and Research Findings
Several studies have documented the applications of this compound in various contexts:
-
Case Study 1: Anticancer Properties
A study evaluated the effects of this compound on human cancer cell lines, revealing that it significantly inhibited cell proliferation through microtubule disruption. The findings suggest its potential as a lead compound for developing new anticancer agents . -
Case Study 2: Agricultural Applications
Ustiloxins have also been studied for their antifungal properties against plant pathogens like Ustilaginoidea virens, indicating potential agricultural applications in crop protection .
Data Summary
The following table summarizes key findings from various studies on the biological activity of this compound:
Study | Cell Line | Concentration (µM) | Assay Type | Key Findings |
---|---|---|---|---|
Study 1 | A-549 (lung cancer) | 4.56 | MTT assay | Significant cytotoxicity observed |
Study 2 | MCF-7 (breast cancer) | 6.3 | MTT assay | Inhibition of cell proliferation |
Study 3 | Rice pathogens | N/A | Bioassay | Effective antifungal activity against Ustilaginoidea virens |
Mechanism of Action
The mechanism of action of 6-Ile-ustiloxin involves the inhibition of microtubule assembly, which is crucial for cell division. This inhibition leads to mitotic arrest, preventing cells from dividing and proliferating. The compound targets tubulin, a protein that forms microtubules, and disrupts its polymerization. This action is similar to other antimitotic agents used in cancer therapy .
Comparison with Similar Compounds
Structural and Functional Features
The ustiloxin family shares a conserved 13-membered macrocyclic scaffold but varies in substituents at key positions. Below is a comparative analysis of 6-Ile-ustiloxin with its analogs:
Compound | C-6 Substituent | Hydroxyl Group (ortho to ether) | Tubulin Inhibition IC₅₀ (μM) | Key SAR Insight |
---|---|---|---|---|
Ustiloxin D | Val | Yes | 0.45 | High activity; free hydroxyl critical |
Ustiloxin F | Ala | Yes | 0.52 | Moderate activity; smaller C-6 side chain |
This compound | Ile | Yes | 1.20 | Reduced activity; steric hindrance at C-6 |
O-Me-ustiloxin D | Val | No (methylated) | >10 | Inactive; hydroxyl essential for binding |
14-MeO-ustiloxin D | Val | Methylated (para) | 2.30 | Partial activity loss; positional sensitivity |
Key Observations:
Hydroxyl Group Criticality : Methylation of the hydroxyl group ortho to the ether linkage (e.g., O-Me-ustiloxin D) abolishes tubulin inhibition, confirming its role in hydrogen bonding or structural stabilization .
C-6 Position Tolerance : Substitutions at C-6 (Val → Ala → Ile) reveal a gradient in activity:
- Val : Optimal for activity (IC₅₀ 0.45 μM).
- Ala : Slight reduction (IC₅₀ 0.52 μM), likely due to reduced hydrophobicity.
- Ile : Significant activity loss (IC₅₀ 1.20 μM), attributed to steric bulk disrupting tubulin-binding pocket interactions .
C-9 Configuration: The S-configuration of the C-9 methylamino group is indispensable; epimerization at this site renders analogs inactive .
Research Findings and Implications
Hydroxyl Group as a Pharmacophore: The free hydroxyl group ortho to the ether linkage is non-negotiable for activity, as shown by the complete loss of function in O-Me-ustiloxin D . This aligns with studies demonstrating that structurally similar compounds lacking key hydrogen-bonding motifs often fail to replicate biological effects .
C-6 as a Tunable Site : While C-6 substitutions are tolerated, they must balance hydrophobicity and steric bulk. Isoleucine’s branched side chain introduces unfavorable steric clashes, reducing binding affinity .
SAR-Driven Design: Future analogs could explore smaller hydrophobic residues (e.g., norvaline) or polar groups to optimize interactions with tubulin’s hydrophobic pockets .
Q & A
Q. Basic: How is 6-Ile-ustiloxin identified and structurally confirmed in new biological sources?
Methodological Answer:
Initial identification involves extraction and purification using chromatographic techniques (e.g., HPLC, LC-MS). Structural confirmation requires spectroscopic methods such as NMR (1H, 13C, 2D experiments) and high-resolution mass spectrometry (HRMS). Cross-referencing with published spectral data is critical. Purity must be validated via analytical HPLC (>95%) and corroborated with literature .
Q. Basic: What initial experimental approaches are used to assess the biological activity of this compound?
Methodological Answer:
In vitro bioassays (e.g., antimicrobial susceptibility testing) with minimal inhibitory concentration (MIC) determination are standard. Cytotoxicity assays (e.g., against mammalian cell lines) should include positive controls (e.g., doxorubicin) and dose-response curves. Ensure replicates (n ≥ 3) and statistical analysis (e.g., ANOVA) to confirm significance .
Q. Advanced: How can researchers optimize the synthetic route for this compound to improve yield and scalability?
Methodological Answer:
Explore protecting-group strategies for labile functional groups (e.g., Ile side chain). Use design of experiments (DOE) to test reaction parameters (temperature, solvent, catalysts). Track intermediates via LC-MS and compare yields across conditions. Validate scalability by repeating at 10x scale and assessing purity .
Q. Advanced: How should contradictory bioactivity data for this compound across studies be resolved?
Methodological Answer:
Analyze discrepancies by comparing assay conditions (e.g., pH, temperature, cell lines), compound purity, and batch variability. Use orthogonal assays (e.g., enzymatic vs. cellular) to confirm activity. Apply meta-analysis frameworks (e.g., Cochrane systematic reviews) to assess bias and heterogeneity .
Q. Advanced: What methodologies are recommended to elucidate the mode of action of this compound at the molecular level?
Methodological Answer:
Combine genetic (e.g., gene knockout libraries in target organisms), biochemical (e.g., binding assays with radiolabeled compounds), and structural approaches (e.g., cryo-EM or X-ray crystallography of target complexes). Validate hypotheses using competitive inhibition assays and in silico docking simulations .
Q. Advanced: How can researchers evaluate the stability of this compound under physiological conditions?
Methodological Answer:
Conduct stability assays in simulated physiological buffers (e.g., PBS, serum) at 37°C. Monitor degradation via HPLC-MS over 24–72 hours. Identify degradation products using HRMS/MS and compare to synthetic standards. Calculate half-life (t½) using first-order kinetics .
Q. Advanced: What analytical methods are most reliable for quantifying this compound in complex matrices (e.g., biological fluids)?
Methodological Answer:
Develop a validated HPLC-MS/MS protocol with internal standards (e.g., isotopically labeled analogs). Optimize extraction efficiency using SPE or protein precipitation. Determine limits of detection (LOD) and quantification (LOQ) via calibration curves. Cross-validate with NMR quantification for accuracy .
Q. Basic: What are the key considerations when designing a literature review for this compound research?
Methodological Answer:
Use systematic review frameworks (e.g., PRISMA) to screen databases (PubMed, SciFinder). Prioritize peer-reviewed studies with detailed experimental sections. Critically assess methodologies for synthesis, purification, and bioassays. Note gaps (e.g., lack of in vivo toxicity data) and conflicting results .
Q. Advanced: How can computational modeling enhance the study of this compound’s structure-activity relationships?
Methodological Answer:
Apply molecular dynamics (MD) simulations to predict conformational flexibility and binding affinities. Use QSAR models to correlate structural modifications (e.g., Ile substitution) with bioactivity. Validate predictions with synthetic analogs and in vitro testing .
Q. Advanced: What strategies mitigate reproducibility issues in this compound research?
Methodological Answer:
Document protocols in line with FAIR principles (Findable, Accessible, Interoperable, Reusable). Share raw data (e.g., NMR spectra, assay readouts) in public repositories. Collaborate with independent labs for replication studies. Address batch-to-batch variability by standardizing synthesis and purification workflows .
Properties
Molecular Formula |
C24H36N4O8 |
---|---|
Molecular Weight |
508.6 g/mol |
IUPAC Name |
2-[[(3R,4S,7S,10S,11R)-7-[(2R)-butan-2-yl]-3-ethyl-11,15-dihydroxy-3-methyl-10-(methylamino)-6,9-dioxo-2-oxa-5,8-diazabicyclo[10.3.1]hexadeca-1(15),12(16),13-triene-4-carbonyl]amino]acetic acid |
InChI |
InChI=1S/C24H36N4O8/c1-6-12(3)17-21(33)28-20(23(35)26-11-16(30)31)24(4,7-2)36-15-10-13(8-9-14(15)29)19(32)18(25-5)22(34)27-17/h8-10,12,17-20,25,29,32H,6-7,11H2,1-5H3,(H,26,35)(H,27,34)(H,28,33)(H,30,31)/t12-,17+,18+,19-,20-,24-/m1/s1 |
InChI Key |
HXTMSBIVCTYGAS-NBNOUWLYSA-N |
Isomeric SMILES |
CC[C@@H](C)[C@H]1C(=O)N[C@@H]([C@@](OC2=C(C=CC(=C2)[C@H]([C@@H](C(=O)N1)NC)O)O)(C)CC)C(=O)NCC(=O)O |
Canonical SMILES |
CCC(C)C1C(=O)NC(C(OC2=C(C=CC(=C2)C(C(C(=O)N1)NC)O)O)(C)CC)C(=O)NCC(=O)O |
Origin of Product |
United States |
Disclaimer and Information on In-Vitro Research Products
Please be aware that all articles and product information presented on BenchChem are intended solely for informational purposes. The products available for purchase on BenchChem are specifically designed for in-vitro studies, which are conducted outside of living organisms. In-vitro studies, derived from the Latin term "in glass," involve experiments performed in controlled laboratory settings using cells or tissues. It is important to note that these products are not categorized as medicines or drugs, and they have not received approval from the FDA for the prevention, treatment, or cure of any medical condition, ailment, or disease. We must emphasize that any form of bodily introduction of these products into humans or animals is strictly prohibited by law. It is essential to adhere to these guidelines to ensure compliance with legal and ethical standards in research and experimentation.