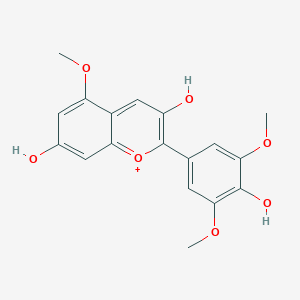
Capensinidin
Overview
Description
Capensinidin is an O-methylated anthocyanidin, a type of water-soluble plant dye that exhibits a blue-red color. It is a 5-methoxy analog of malvidin and has been isolated from the plant Plumbago capensis
Mechanism of Action
Target of Action
Capensinidin is an O-methylated anthocyanidin . Anthocyanidins are a type of flavonoid, a class of compounds with potential antioxidant and health benefits. They are commonly found in fruits and vegetables, and contribute to the blue-red color of many fruits and flowers . .
Biochemical Pathways
Anthocyanidins like this compound are known to be involved in the regulation of oxidative stress and inflammation, suggesting that they may affect pathways related to these processes .
Pharmacokinetics
As a water-soluble compound , it is expected to have different pharmacokinetic properties compared to fat-soluble compounds. The impact of these properties on this compound’s bioavailability is currently unknown.
Result of Action
Anthocyanidins like this compound are known to have potential antioxidant and anti-inflammatory effects , suggesting that this compound may have similar effects.
Biochemical Analysis
Biochemical Properties
Capensinidin, being an anthocyanidin, plays a significant role in plant biochemistry. Anthocyanidins are plant pigments that contribute to the colors of many flowers, fruits, and leaves
Cellular Effects
They can interact with various cellular processes and potentially influence cell function, including impacts on cell signaling pathways, gene expression, and cellular metabolism .
Metabolic Pathways
This compound, as an anthocyanidin, is part of the flavonoid biosynthesis pathway This pathway involves several enzymes and cofactors
Preparation Methods
Synthetic Routes and Reaction Conditions: Capensinidin can be synthesized through the methylation of anthocyanidins. The process involves the use of methylating agents such as dimethyl sulfate or methyl iodide under basic conditions. The reaction typically requires a solvent like methanol or ethanol and a base such as sodium hydroxide or potassium carbonate to facilitate the methylation process.
Industrial Production Methods: Industrial production of this compound involves the extraction of the compound from natural sources like Plumbago capensis. The extraction process includes maceration of the plant material in a suitable solvent, followed by purification steps such as filtration, evaporation, and crystallization to isolate the pure compound .
Chemical Reactions Analysis
Types of Reactions: Capensinidin undergoes various chemical reactions, including:
Oxidation: this compound can be oxidized to form quinonoid intermediates, which can further react to form different oxidation products.
Reduction: Reduction of this compound can lead to the formation of leucoanthocyanidins, which are colorless compounds.
Substitution: The hydroxyl groups in this compound can undergo substitution reactions with different reagents to form derivatives with altered properties.
Common Reagents and Conditions:
Oxidation: Common oxidizing agents include hydrogen peroxide and potassium permanganate.
Reduction: Reducing agents such as sodium borohydride and lithium aluminum hydride are used.
Substitution: Reagents like acyl chlorides and alkyl halides are used for substitution reactions.
Major Products Formed:
Oxidation Products: Quinonoid intermediates and other oxidized derivatives.
Reduction Products: Leucoanthocyanidins.
Substitution Products: Various substituted derivatives with modified functional groups.
Scientific Research Applications
Capensinidin has a wide range of scientific research applications, including:
Chemistry: Used as a pH indicator due to its color change properties in different pH environments.
Biology: Studied for its antioxidant properties and potential role in protecting cells from oxidative stress.
Medicine: Investigated for its potential anti-inflammatory and anticancer properties.
Comparison with Similar Compounds
- Malvidin
- Delphinidin
- Cyanidin
Capensinidin’s unique structure and properties make it a valuable compound for various scientific and industrial applications.
Properties
IUPAC Name |
2-(4-hydroxy-3,5-dimethoxyphenyl)-5-methoxychromenylium-3,7-diol | |
---|---|---|
Source | PubChem | |
URL | https://pubchem.ncbi.nlm.nih.gov | |
Description | Data deposited in or computed by PubChem | |
InChI |
InChI=1S/C18H16O7/c1-22-13-6-10(19)7-14-11(13)8-12(20)18(25-14)9-4-15(23-2)17(21)16(5-9)24-3/h4-8H,1-3H3,(H2-,19,20,21)/p+1 | |
Source | PubChem | |
URL | https://pubchem.ncbi.nlm.nih.gov | |
Description | Data deposited in or computed by PubChem | |
InChI Key |
GYLVPQXQQPMCKK-UHFFFAOYSA-O | |
Source | PubChem | |
URL | https://pubchem.ncbi.nlm.nih.gov | |
Description | Data deposited in or computed by PubChem | |
Canonical SMILES |
COC1=CC(=CC(=C1O)OC)C2=C(C=C3C(=CC(=CC3=[O+]2)O)OC)O | |
Source | PubChem | |
URL | https://pubchem.ncbi.nlm.nih.gov | |
Description | Data deposited in or computed by PubChem | |
Molecular Formula |
C18H17O7+ | |
Source | PubChem | |
URL | https://pubchem.ncbi.nlm.nih.gov | |
Description | Data deposited in or computed by PubChem | |
DSSTOX Substance ID |
DTXSID00331604 | |
Record name | Capensinidin | |
Source | EPA DSSTox | |
URL | https://comptox.epa.gov/dashboard/DTXSID00331604 | |
Description | DSSTox provides a high quality public chemistry resource for supporting improved predictive toxicology. | |
Molecular Weight |
345.3 g/mol | |
Source | PubChem | |
URL | https://pubchem.ncbi.nlm.nih.gov | |
Description | Data deposited in or computed by PubChem | |
CAS No. |
19077-85-1 | |
Record name | Capensinidin | |
Source | EPA DSSTox | |
URL | https://comptox.epa.gov/dashboard/DTXSID00331604 | |
Description | DSSTox provides a high quality public chemistry resource for supporting improved predictive toxicology. | |
Retrosynthesis Analysis
AI-Powered Synthesis Planning: Our tool employs the Template_relevance Pistachio, Template_relevance Bkms_metabolic, Template_relevance Pistachio_ringbreaker, Template_relevance Reaxys, Template_relevance Reaxys_biocatalysis model, leveraging a vast database of chemical reactions to predict feasible synthetic routes.
One-Step Synthesis Focus: Specifically designed for one-step synthesis, it provides concise and direct routes for your target compounds, streamlining the synthesis process.
Accurate Predictions: Utilizing the extensive PISTACHIO, BKMS_METABOLIC, PISTACHIO_RINGBREAKER, REAXYS, REAXYS_BIOCATALYSIS database, our tool offers high-accuracy predictions, reflecting the latest in chemical research and data.
Strategy Settings
Precursor scoring | Relevance Heuristic |
---|---|
Min. plausibility | 0.01 |
Model | Template_relevance |
Template Set | Pistachio/Bkms_metabolic/Pistachio_ringbreaker/Reaxys/Reaxys_biocatalysis |
Top-N result to add to graph | 6 |
Feasible Synthetic Routes
Q1: What is unique about the structure of capensinidin compared to other anthocyanidins, and how does this impact its properties?
A1: this compound is a unique anthocyanidin because it possesses two methoxy groups at the 5 and 7 positions of its A-ring, resulting in no free hydroxyl groups in these positions []. This structural feature differentiates it from most other naturally occurring anthocyanidins, which typically have at least one free hydroxyl group in the A-ring. The presence of methoxy groups instead of hydroxyl groups prevents the formation of tautomeric quinonoidal bases (anhydrobases), which are commonly observed in other anthocyanins []. This structural difference might influence this compound's color, stability, and potential biological activity compared to other anthocyanidins.
Q2: What potential therapeutic benefits has this compound demonstrated in computational studies, and what mechanisms might be responsible for these effects?
A2: In silico docking studies have suggested that this compound may be a promising lead compound for developing treatments for neurodegenerative diseases like Parkinson's disease []. These studies showed that this compound exhibits favorable binding energies to target proteins involved in Parkinson's disease pathogenesis, comparable to known standard reference compounds []. While the exact mechanisms of action remain to be elucidated, it is plausible that this compound's ability to interact with these specific protein targets could contribute to its potential neuroprotective effects. Further research, including in vitro and in vivo studies, is necessary to confirm these initial computational findings and explore the therapeutic potential of this compound for neurodegenerative diseases.
Disclaimer and Information on In-Vitro Research Products
Please be aware that all articles and product information presented on BenchChem are intended solely for informational purposes. The products available for purchase on BenchChem are specifically designed for in-vitro studies, which are conducted outside of living organisms. In-vitro studies, derived from the Latin term "in glass," involve experiments performed in controlled laboratory settings using cells or tissues. It is important to note that these products are not categorized as medicines or drugs, and they have not received approval from the FDA for the prevention, treatment, or cure of any medical condition, ailment, or disease. We must emphasize that any form of bodily introduction of these products into humans or animals is strictly prohibited by law. It is essential to adhere to these guidelines to ensure compliance with legal and ethical standards in research and experimentation.