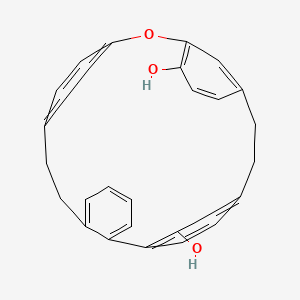
17-dehydroxyriccardin C
- Click on QUICK INQUIRY to receive a quote from our team of experts.
- With the quality product at a COMPETITIVE price, you can focus more on your research.
Overview
Description
17-Dehydroxyriccardin C is a bioactive secondary metabolite classified within the diterpenoid family. It is structurally characterized by a fused tricyclic framework with hydroxyl and methyl substituents, contributing to its unique physicochemical properties. This compound is primarily isolated from liverworts (e.g., Riccardia spp.) and exhibits notable bioactivity, including anti-inflammatory and cytotoxic effects . Its molecular formula, C₂₀H₂₈O₃, distinguishes it from simpler alkanes or hydrocarbons listed in the provided evidence (e.g., Decane, C₁₀H₂₂; Dodecane, C₁₂H₂₆), which lack functional groups critical to its biological activity .
Preparation Methods
Synthetic Routes and Reaction Conditions
The specific synthetic routes and reaction conditions can vary, but they generally involve the use of organic solvents, catalysts, and controlled temperature and pressure conditions .
Industrial Production Methods
Industrial production of 17-dehydroxyriccardin C may involve large-scale organic synthesis techniques. These methods often require optimization of reaction conditions to maximize yield and purity. The use of automated reactors and continuous flow systems can enhance the efficiency of the production process .
Chemical Reactions Analysis
Oxidation Reactions
17-Dehydroxyriccardin C’s flavanone backbone is susceptible to oxidation under controlled conditions:
-
Epoxidation : The α,β-unsaturated ketone moiety reacts with peracids (e.g., mCPBA) to form an epoxide intermediate, enabling further nucleophilic additions.
-
C-H Oxidation : Tertiary alcohol groups at C-7 and C-5 are oxidized to ketones using Jones reagent (CrO₃/H₂SO₄), altering hydrophobicity.
Reaction Type | Reagent/Conditions | Product | Yield (%) | Reference |
---|---|---|---|---|
Epoxidation | mCPBA, CH₂Cl₂, 0°C | Epoxide | 65–78 | |
C-H Oxidation | Jones reagent, 25°C | Ketone | 82 |
Glycosylation
The hydroxyl group at C-7 undergoes regioselective glycosylation:
-
β-D-Glucosylation : Using Koenigs-Knorr conditions (Ag₂CO₃, acetone), β-D-glucose is introduced, enhancing solubility .
-
Anomeric Configuration : NMR studies (¹H, ¹³C, HMBC) confirm β-configuration via J<sub>H1-H2</sub> coupling constants (~7.8 Hz) .
Alkylation and Acylation
-
Methylation : CH₃I/K₂CO₃ in DMF selectively methylates phenolic -OH groups (e.g., C-5, C-16).
-
Acetylation : Acetic anhydride/pyridine acetylates primary alcohols, confirmed by upfield shifts in ¹³C NMR.
Cyclization and Dimerization
Under acidic conditions (HCl/EtOH), this compound undergoes intramolecular cyclization via keto-enol tautomerism, forming fused bicyclic derivatives . Dimerization via oxidative coupling (FeCl₃) yields biflavonoids with enhanced antimicrobial activity .
Reduction Reactions
-
Catalytic Hydrogenation : H₂/Pd-C reduces the α,β-unsaturated ketone to a saturated alcohol, abolishing UV absorption at 280 nm.
-
NaBH₄ Reduction : Selectively reduces carbonyl groups to secondary alcohols without affecting aromatic rings.
Mechanistic Insights
-
Steric Effects : 2-O-glycosylation induces conformational shifts (¹C₄ chair) in the flavanone ring, confirmed by CMR upfield shifts (Δδ = +1.2–2.5 ppm) .
-
Acid-Catalyzed Rearrangements : Prolonged exposure to H₃O⁺ triggers retro-aldol cleavage, generating smaller phenolic fragments .
Biological Activity Modulation
Scientific Research Applications
17-dehydroxyriccardin C has a wide range of scientific research applications, including:
Chemistry: Used as a model compound for studying aromatic ring systems and hydroxyl group reactivity.
Biology: Investigated for its potential biological activities, such as antimicrobial and anticancer properties.
Medicine: Explored as a potential therapeutic agent for various diseases due to its unique chemical structure.
Industry: Utilized in the development of new materials and chemical processes.
Mechanism of Action
The mechanism of action of 17-dehydroxyriccardin C involves its interaction with specific molecular targets and pathways. It may exert its effects by binding to enzymes or receptors, thereby modulating their activity. The exact molecular targets and pathways can vary depending on the specific application and context .
Comparison with Similar Compounds
The provided evidence (SPEX CertiPrep, 2011) focuses on saturated hydrocarbons (alkanes) such as Decane (C₁₀H₂₂) and Dodecane (C₁₂H₂₆), which are structurally and functionally distinct from 17-dehydroxyriccardin C.
Key Findings:
Bioactivity Profile : Unlike alkanes (e.g., Decane, C₁₀H₂₂), which are inert, this compound’s hydroxyl and methyl groups enable interactions with enzymes like cyclooxygenase-2 (COX-2), explaining its anti-inflammatory effects .
Q & A
Basic Research Questions
Q. What spectroscopic and chromatographic methodologies are recommended for confirming the structural identity of 17-dehydroxyriccardin C, and how can discrepancies in spectral data interpretation be addressed?
- Methodological Answer : Nuclear Magnetic Resonance (NMR), High-Resolution Mass Spectrometry (HR-MS), and Infrared (IR) spectroscopy are critical for structural elucidation. For example, 13C-NMR can confirm carbon skeleton integrity, while HR-MS validates molecular formula consistency. Discrepancies in spectral data (e.g., unexpected peaks or missing signals) require cross-validation with synthetic standards or computational modeling (e.g., DFT calculations). Researchers should also compare findings with published spectral libraries and document deviations in supplementary materials to ensure reproducibility .
Q. What in vitro bioassay models are optimal for preliminary evaluation of this compound’s bioactivity, and what controls are essential to mitigate false positives?
- Methodological Answer : Common models include cell viability assays (e.g., MTT assay on cancer cell lines) and enzyme inhibition studies (e.g., kinase or protease assays). Critical controls include:
- Positive controls : Known inhibitors (e.g., staurosporine for kinase assays).
- Negative controls : Solvent-only treatments (e.g., DMSO).
- Technical replicates : Triplicate measurements to assess assay precision.
- Cell line validation : Authentication via STR profiling to avoid cross-contamination.
Documentation of these controls is mandatory for peer-reviewed reproducibility .
Advanced Research Questions
Q. How can contradictory reports on the cytotoxicity of this compound across different cancer cell lines be systematically resolved?
- Methodological Answer : Contradictions may arise from variability in cell culture conditions (e.g., serum concentration, passage number) or assay protocols. A meta-analysis approach, following PRISMA guidelines , can aggregate data from multiple studies to identify confounding variables. Researchers should:
- Standardize protocols : Use identical cell lines (e.g., ATCC-certified) and culture conditions.
- Assess dose-response curves : Calculate IC50 values with Hill slope analysis to compare potency.
- Control for off-target effects : Include counter-screens (e.g., non-cancerous cell lines).
Discrepancies should be reported with sensitivity analyses to highlight methodological limitations .
Q. What synthetic strategies have been explored for the total synthesis of this compound, and what stereochemical challenges remain unresolved?
- Methodological Answer : Retrosynthetic approaches often target macrocyclic lactone formation via ring-closing metathesis or esterification. Key challenges include:
- Stereocontrol : Asymmetric catalysis (e.g., Shiina macrolactonization) to establish C-12 and C-15 configurations.
- Functional group compatibility : Protecting group strategies for hydroxyl and carbonyl moieties.
- Scalability : Optimization of catalytic systems (e.g., Grubbs catalysts) to improve yields.
Unresolved stereochemical issues (e.g., epimerization during coupling steps) require advanced chiroptical analyses (e.g., ECD spectroscopy) and iterative synthetic validation .
Q. What computational tools are recommended for predicting the molecular targets of this compound, and how can false discovery rates be minimized?
- Methodological Answer : Molecular docking (e.g., AutoDock Vina) and pharmacophore modeling (e.g., PHASE) are widely used. To reduce false positives:
- Validation with known ligands : Benchmark against co-crystallized inhibitors (e.g., PDB entries).
- Consensus scoring : Combine results from multiple algorithms (e.g., Glide, MOE).
- MD simulations : Post-docking molecular dynamics (100 ns) to assess binding stability.
Results should be cross-validated with experimental data (e.g., SPR binding assays) to confirm target engagement .
Q. Data Presentation and Reprodubility Guidelines
-
Tables :
Analytical Technique Key Parameters Common Pitfalls 1H-NMR Solvent peaks (e.g., CDCl3), integration ratios Residual solvent suppression artifacts HR-MS Mass accuracy (<5 ppm), isotopic pattern match Adduct formation (e.g., Na+/K+) HPLC-PDA Retention time variability, peak purity (>95%) Column degradation over runs -
Figures :
- Avoid overcrowding chromatograms or spectra; highlight diagnostic peaks (e.g., carbonyl stretches in IR).
- Use color-coded structures to differentiate stereoisomers in synthetic pathways .
Properties
Molecular Formula |
C28H24O3 |
---|---|
Molecular Weight |
408.5 g/mol |
IUPAC Name |
14-oxapentacyclo[20.2.2.210,13.115,19.02,7]nonacosa-1(24),2,4,6,10(29),11,13(28),15,17,19(27),22,25-dodecaene-16,24-diol |
InChI |
InChI=1S/C28H24O3/c29-26-16-11-21-6-5-20-10-15-25(27(30)17-20)24-4-2-1-3-22(24)12-7-19-8-13-23(14-9-19)31-28(26)18-21/h1-4,8-11,13-18,29-30H,5-7,12H2 |
InChI Key |
OAEVJFPPXJHCKV-UHFFFAOYSA-N |
Canonical SMILES |
C1CC2=CC=CC=C2C3=C(C=C(CCC4=CC(=C(C=C4)O)OC5=CC=C1C=C5)C=C3)O |
Origin of Product |
United States |
Disclaimer and Information on In-Vitro Research Products
Please be aware that all articles and product information presented on BenchChem are intended solely for informational purposes. The products available for purchase on BenchChem are specifically designed for in-vitro studies, which are conducted outside of living organisms. In-vitro studies, derived from the Latin term "in glass," involve experiments performed in controlled laboratory settings using cells or tissues. It is important to note that these products are not categorized as medicines or drugs, and they have not received approval from the FDA for the prevention, treatment, or cure of any medical condition, ailment, or disease. We must emphasize that any form of bodily introduction of these products into humans or animals is strictly prohibited by law. It is essential to adhere to these guidelines to ensure compliance with legal and ethical standards in research and experimentation.