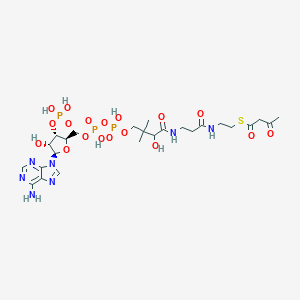
acetoacetyl-CoA
Overview
Description
Preparation Methods
Synthetic Routes and Reaction Conditions: Acetoacetyl-CoA is synthesized from acetyl-CoA through a Claisen condensation reaction. This reaction involves the enolate form of one acetyl-CoA molecule reacting with another acetyl-CoA molecule, catalyzed by thiolase enzymes .
Industrial Production Methods: Industrial production of this compound typically involves biotechnological methods using genetically engineered microorganisms. These microorganisms are designed to overproduce the necessary enzymes, such as thiolase, to catalyze the formation of this compound from acetyl-CoA .
Types of Reactions:
Reduction: It can be reduced to ®-3-hydroxybutyryl-CoA by NADPH-dependent acetoacetyl-coenzyme A reductase.
Common Reagents and Conditions:
Oxidation: NADPH and acetoacetyl-coenzyme A reductase are commonly used in oxidation reactions.
Reduction: NADPH and acetoacetyl-coenzyme A reductase are also used in reduction reactions.
Substitution: Acetyl-CoA and HMG-CoA synthase are used in substitution reactions to form HMG-CoA.
Major Products:
®-3-hydroxybutyryl-CoA: Formed through reduction reactions.
HMG-CoA: Formed through substitution reactions in the mevalonate pathway.
Scientific Research Applications
Metabolic Pathways
Acetoacetyl-CoA in Lipid Metabolism
this compound plays a crucial role in the synthesis of ketone bodies and fatty acids. It is synthesized from acetyl-CoA and is a precursor to HMG-CoA in the mevalonate pathway, which is essential for cholesterol and terpenoid biosynthesis . The enzyme this compound synthetase (AACS) catalyzes the conversion of acetoacetate to this compound, facilitating the anabolic utilization of ketone bodies for de novo lipid synthesis .
Table 1: Key Enzymes Involved in this compound Metabolism
Enzyme | Function | Substrates |
---|---|---|
This compound Synthetase | Converts acetoacetate to this compound | Acetoacetate |
This compound Thiolase | Cleaves this compound into acetyl-CoA | This compound |
HMG-CoA Synthase | Converts this compound to HMG-CoA | This compound, Acetyl-CoA |
Biotechnological Applications
Polyhydroxyalkanoates (PHAs) Production
this compound is a precursor for the biosynthesis of polyhydroxyalkanoates, biodegradable polymers produced by microbial fermentation. Engineering microbial strains to enhance this compound production can lead to increased yields of PHAs, which have applications in bioplastics and tissue engineering .
Case Study: Engineering E. coli for PHA Production
Research has demonstrated that by expressing specific enzymes such as this compound reductase in Escherichia coli, researchers can significantly increase PHAs accumulation. This approach involves manipulating metabolic pathways to optimize the flow of substrates towards this compound .
Clinical Implications
Mitochondrial Disorders
Deficiencies in enzymes involved in this compound metabolism can lead to mitochondrial disorders. For example, mitochondrial this compound thiolase deficiency has been documented, affecting patients' ability to metabolize ketone bodies effectively . Clinical management often involves dietary adjustments to manage metabolic crises.
Table 2: Clinical Cases of this compound Related Disorders
Patient ID | Age at Diagnosis | Symptoms | Treatment |
---|---|---|---|
GK45 | 15 months | Ketoacidotic episodes | Moderate protein-restricted diet |
GK47 | 19 months | Severe ketoacidotic episodes | L-carnitine supplementation |
Pharmaceutical Applications
This compound's role as a substrate for various biosynthetic pathways makes it a target for drug development. Its derivatives are explored for their potential as therapeutic agents due to their involvement in lipid metabolism and energy production.
Research Findings on AACS
Recent studies have highlighted AACS as a potential target for therapeutic interventions aimed at metabolic disorders, suggesting that modulation of this enzyme could influence lipid synthesis pathways beneficially .
Mechanism of Action
Acetoacetyl-CoA exerts its effects through its role as an intermediate in various metabolic pathways. It is involved in the synthesis of HMG-CoA in the mevalonate pathway, which is crucial for cholesterol biosynthesis . Additionally, it participates in the ketogenesis pathway, where it is converted to acetoacetate and subsequently to other ketone bodies . The molecular targets and pathways involved include thiolase enzymes, HMG-CoA synthase, and NADPH-dependent acetoacetyl-coenzyme A reductase .
Comparison with Similar Compounds
Acetyl-CoA: A precursor to acetoacetyl-CoA, involved in numerous metabolic pathways.
HMG-CoA: Formed from this compound in the mevalonate pathway.
®-3-hydroxybutyryl-CoA: Formed from the reduction of this compound.
Uniqueness: this compound is unique due to its dual role in both the mevalonate pathway for cholesterol biosynthesis and the ketogenesis pathway for energy production . Its ability to participate in multiple types of reactions, including oxidation, reduction, and substitution, further highlights its versatility and importance in metabolic processes .
Biological Activity
Acetoacetyl-CoA is a pivotal intermediate in various metabolic pathways, particularly in lipid metabolism and ketogenesis. This article explores its biological activity, mechanisms of action, and implications in health and disease, supported by diverse research findings and case studies.
Overview of this compound
This compound is synthesized from two molecules of acetyl-CoA through a Claisen condensation reaction catalyzed by this compound thiolase. It serves as a precursor for several critical biochemical pathways, including the synthesis of cholesterol via the mevalonate pathway and the production of ketone bodies during periods of fasting or low carbohydrate intake .
Key Biological Functions
- Cholesterol Biosynthesis :
- Ketogenesis :
- Lipid Synthesis :
Enzymatic Reactions Involving this compound
Enzyme | Reaction | Product |
---|---|---|
This compound thiolase | Condensation of acetyl-CoA | This compound |
HMG-CoA synthase | Conversion of this compound to HMG-CoA | HMG-CoA |
AACS | Conversion of acetoacetate to this compound | This compound |
1. This compound and Neurological Development
Research indicates that this compound plays a crucial role in neuronal development by providing essential lipids for myelination and synaptic function. High expression levels of AACS have been observed in the hippocampus, suggesting its involvement in cognitive functions .
2. Genetic Disorders Related to this compound Metabolism
Mutations in genes encoding enzymes involved in this compound metabolism can lead to metabolic disorders:
- Beta-Ketothiolase Deficiency : A rare disorder characterized by an inability to metabolize ketone bodies properly, leading to accumulation of this compound and related metabolites .
- Mitochondrial HMG-CoA Synthase Deficiency : This condition results from mutations affecting ketone body metabolism, causing severe hypoglycemia and neurological symptoms due to impaired energy production from fats .
3. Engineering this compound Reductases
Recent studies have focused on engineering this compound reductases to enhance the production of polyhydroxyalkanoates (PHAs), biodegradable plastics produced by bacteria. These engineered enzymes exhibit improved efficiency for industrial applications, highlighting the biotechnological potential of manipulating this compound pathways .
Implications for Health and Disease
The biological activity of this compound has significant implications for metabolic health:
- Ketogenic Diets : The elevation of this compound levels during ketogenic diets can lead to increased production of ketone bodies, which may benefit individuals with epilepsy or metabolic disorders but can also pose risks if not managed properly .
- Cholesterol Management : Understanding the role of this compound in cholesterol biosynthesis may provide insights into therapeutic targets for hyperlipidemia and cardiovascular diseases .
Properties
IUPAC Name |
S-[2-[3-[[(2R)-4-[[[(2R,3S,4R,5R)-5-(6-aminopurin-9-yl)-4-hydroxy-3-phosphonooxyoxolan-2-yl]methoxy-hydroxyphosphoryl]oxy-hydroxyphosphoryl]oxy-2-hydroxy-3,3-dimethylbutanoyl]amino]propanoylamino]ethyl] 3-oxobutanethioate | |
---|---|---|
Source | PubChem | |
URL | https://pubchem.ncbi.nlm.nih.gov | |
Description | Data deposited in or computed by PubChem | |
InChI |
InChI=1S/C25H40N7O18P3S/c1-13(33)8-16(35)54-7-6-27-15(34)4-5-28-23(38)20(37)25(2,3)10-47-53(44,45)50-52(42,43)46-9-14-19(49-51(39,40)41)18(36)24(48-14)32-12-31-17-21(26)29-11-30-22(17)32/h11-12,14,18-20,24,36-37H,4-10H2,1-3H3,(H,27,34)(H,28,38)(H,42,43)(H,44,45)(H2,26,29,30)(H2,39,40,41)/t14-,18-,19-,20+,24-/m1/s1 | |
Source | PubChem | |
URL | https://pubchem.ncbi.nlm.nih.gov | |
Description | Data deposited in or computed by PubChem | |
InChI Key |
OJFDKHTZOUZBOS-CITAKDKDSA-N | |
Source | PubChem | |
URL | https://pubchem.ncbi.nlm.nih.gov | |
Description | Data deposited in or computed by PubChem | |
Canonical SMILES |
CC(=O)CC(=O)SCCNC(=O)CCNC(=O)C(C(C)(C)COP(=O)(O)OP(=O)(O)OCC1C(C(C(O1)N2C=NC3=C(N=CN=C32)N)O)OP(=O)(O)O)O | |
Source | PubChem | |
URL | https://pubchem.ncbi.nlm.nih.gov | |
Description | Data deposited in or computed by PubChem | |
Isomeric SMILES |
CC(=O)CC(=O)SCCNC(=O)CCNC(=O)[C@@H](C(C)(C)COP(=O)(O)OP(=O)(O)OC[C@@H]1[C@H]([C@H]([C@@H](O1)N2C=NC3=C(N=CN=C32)N)O)OP(=O)(O)O)O | |
Source | PubChem | |
URL | https://pubchem.ncbi.nlm.nih.gov | |
Description | Data deposited in or computed by PubChem | |
Molecular Formula |
C25H40N7O18P3S | |
Source | PubChem | |
URL | https://pubchem.ncbi.nlm.nih.gov | |
Description | Data deposited in or computed by PubChem | |
DSSTOX Substance ID |
DTXSID30931292 | |
Record name | S-Acetoacetylcoenzyme A | |
Source | EPA DSSTox | |
URL | https://comptox.epa.gov/dashboard/DTXSID30931292 | |
Description | DSSTox provides a high quality public chemistry resource for supporting improved predictive toxicology. | |
Molecular Weight |
851.6 g/mol | |
Source | PubChem | |
URL | https://pubchem.ncbi.nlm.nih.gov | |
Description | Data deposited in or computed by PubChem | |
Physical Description |
Solid | |
Record name | Acetoacetyl-CoA | |
Source | Human Metabolome Database (HMDB) | |
URL | http://www.hmdb.ca/metabolites/HMDB0001484 | |
Description | The Human Metabolome Database (HMDB) is a freely available electronic database containing detailed information about small molecule metabolites found in the human body. | |
Explanation | HMDB is offered to the public as a freely available resource. Use and re-distribution of the data, in whole or in part, for commercial purposes requires explicit permission of the authors and explicit acknowledgment of the source material (HMDB) and the original publication (see the HMDB citing page). We ask that users who download significant portions of the database cite the HMDB paper in any resulting publications. | |
CAS No. |
1420-36-6 | |
Record name | Acetoacetyl-CoA | |
Source | CAS Common Chemistry | |
URL | https://commonchemistry.cas.org/detail?cas_rn=1420-36-6 | |
Description | CAS Common Chemistry is an open community resource for accessing chemical information. Nearly 500,000 chemical substances from CAS REGISTRY cover areas of community interest, including common and frequently regulated chemicals, and those relevant to high school and undergraduate chemistry classes. This chemical information, curated by our expert scientists, is provided in alignment with our mission as a division of the American Chemical Society. | |
Explanation | The data from CAS Common Chemistry is provided under a CC-BY-NC 4.0 license, unless otherwise stated. | |
Record name | Acetoacetyl coa | |
Source | ChemIDplus | |
URL | https://pubchem.ncbi.nlm.nih.gov/substance/?source=chemidplus&sourceid=0001420366 | |
Description | ChemIDplus is a free, web search system that provides access to the structure and nomenclature authority files used for the identification of chemical substances cited in National Library of Medicine (NLM) databases, including the TOXNET system. | |
Record name | Acetoacetyl-CoA | |
Source | DrugBank | |
URL | https://www.drugbank.ca/drugs/DB03059 | |
Description | The DrugBank database is a unique bioinformatics and cheminformatics resource that combines detailed drug (i.e. chemical, pharmacological and pharmaceutical) data with comprehensive drug target (i.e. sequence, structure, and pathway) information. | |
Explanation | Creative Common's Attribution-NonCommercial 4.0 International License (http://creativecommons.org/licenses/by-nc/4.0/legalcode) | |
Record name | S-Acetoacetylcoenzyme A | |
Source | EPA DSSTox | |
URL | https://comptox.epa.gov/dashboard/DTXSID30931292 | |
Description | DSSTox provides a high quality public chemistry resource for supporting improved predictive toxicology. | |
Record name | S-acetoacetylcoenzyme A | |
Source | European Chemicals Agency (ECHA) | |
URL | https://echa.europa.eu/substance-information/-/substanceinfo/100.014.378 | |
Description | The European Chemicals Agency (ECHA) is an agency of the European Union which is the driving force among regulatory authorities in implementing the EU's groundbreaking chemicals legislation for the benefit of human health and the environment as well as for innovation and competitiveness. | |
Explanation | Use of the information, documents and data from the ECHA website is subject to the terms and conditions of this Legal Notice, and subject to other binding limitations provided for under applicable law, the information, documents and data made available on the ECHA website may be reproduced, distributed and/or used, totally or in part, for non-commercial purposes provided that ECHA is acknowledged as the source: "Source: European Chemicals Agency, http://echa.europa.eu/". Such acknowledgement must be included in each copy of the material. ECHA permits and encourages organisations and individuals to create links to the ECHA website under the following cumulative conditions: Links can only be made to webpages that provide a link to the Legal Notice page. | |
Record name | Acetoacetyl-CoA | |
Source | Human Metabolome Database (HMDB) | |
URL | http://www.hmdb.ca/metabolites/HMDB0001484 | |
Description | The Human Metabolome Database (HMDB) is a freely available electronic database containing detailed information about small molecule metabolites found in the human body. | |
Explanation | HMDB is offered to the public as a freely available resource. Use and re-distribution of the data, in whole or in part, for commercial purposes requires explicit permission of the authors and explicit acknowledgment of the source material (HMDB) and the original publication (see the HMDB citing page). We ask that users who download significant portions of the database cite the HMDB paper in any resulting publications. | |
Retrosynthesis Analysis
AI-Powered Synthesis Planning: Our tool employs the Template_relevance Pistachio, Template_relevance Bkms_metabolic, Template_relevance Pistachio_ringbreaker, Template_relevance Reaxys, Template_relevance Reaxys_biocatalysis model, leveraging a vast database of chemical reactions to predict feasible synthetic routes.
One-Step Synthesis Focus: Specifically designed for one-step synthesis, it provides concise and direct routes for your target compounds, streamlining the synthesis process.
Accurate Predictions: Utilizing the extensive PISTACHIO, BKMS_METABOLIC, PISTACHIO_RINGBREAKER, REAXYS, REAXYS_BIOCATALYSIS database, our tool offers high-accuracy predictions, reflecting the latest in chemical research and data.
Strategy Settings
Precursor scoring | Relevance Heuristic |
---|---|
Min. plausibility | 0.01 |
Model | Template_relevance |
Template Set | Pistachio/Bkms_metabolic/Pistachio_ringbreaker/Reaxys/Reaxys_biocatalysis |
Top-N result to add to graph | 6 |
Feasible Synthetic Routes
Q1: How does acetoacetyl-CoA interact with Hydroxymethylglutaryl-CoA synthase (HMG-CoA synthase) and what are the downstream effects?
A1: this compound acts as a substrate for HMG-CoA synthase, binding to the enzyme's active site. [] This interaction initiates the condensation reaction with acetyl-CoA, ultimately leading to the formation of HMG-CoA, a precursor for cholesterol and isoprenoid biosynthesis. [] Interestingly, this compound also exhibits substrate inhibition of HMG-CoA synthase at higher concentrations, competing with acetyl-CoA. [] This suggests a regulatory role for this compound in cholesterol synthesis. [] Studies in avian enzymes propose that histidine 264 (H264) in HMG-CoA synthase interacts with the carbonyl oxygen of the this compound thioester, potentially anchoring the substrate for catalysis. []
Q2: What role does this compound play in regulating fatty acid oxidation?
A2: this compound can act as a feedback inhibitor of enoyl-CoA hydratase (crotonase), a key enzyme in the fatty acid β-oxidation pathway. [] This inhibition is attributed to the enolate form of this compound, which competes with crotonyl-CoA for the enzyme's active site. [] This interaction highlights a regulatory mechanism where increased this compound levels can downregulate fatty acid oxidation. []
Q3: Can this compound be utilized by pathways other than those involving CoA-transferases?
A3: Research on Desulfococcus biacutus suggests that this compound can be formed from acetone without involving CoA-transferases or CoA ligases. [] This process is proposed to occur via a carboxylation reaction followed by activation to this compound, without a free intermediate. []
Q4: What is the molecular formula and weight of this compound?
A4: While not explicitly stated in the provided research, this compound's molecular formula is C25H40N7O18P3S, and its molecular weight is 851.6 g/mol.
Q5: How can spectroscopic techniques be used to study this compound?
A5: Resonance Raman (RR) spectroscopy has been instrumental in understanding the interaction of this compound with enzymes like medium-chain acyl-CoA dehydrogenase (MCAD). [, ] By analyzing the RR spectra, researchers have confirmed the formation of charge-transfer complexes between the enzyme's flavin cofactor and this compound, providing insights into the substrate activation mechanism. [, ] 13C-NMR spectroscopy has also been utilized to study the changes in the electronic environment of this compound's carbon atoms upon binding to MCAD. [] These studies indicate that this compound adopts an enolate form when bound to the enzyme, suggesting a mechanism for substrate activation. []
Q6: What are the key enzymes that utilize this compound as a substrate?
A6: Several enzymes utilize this compound, playing critical roles in various metabolic pathways. Some examples include:
Q7: How does the kinetic mechanism of this compound thiolase contribute to its regulatory role?
A7: this compound thiolase exhibits a ping-pong kinetic mechanism, involving the formation of a covalent acetyl-enzyme intermediate. [, ] This mechanism, along with the enzyme's susceptibility to inhibition by CoA and this compound, suggests intricate regulation. [, ] In the direction of this compound synthesis, CoA acts as a substrate inhibitor, competing with this compound. [] Conversely, in the direction of this compound cleavage, this compound can exhibit substrate inhibition, particularly in the absence of CoA. [] These regulatory features highlight the enzyme's role in maintaining cellular CoA and acetyl-CoA balance.
Q8: How does potassium ion concentration affect the activity of mitochondrial this compound thiolase (T2)?
A8: T2 displays a unique activation by potassium ions (K+). [] Kinetic studies have shown that increasing K+ concentration enhances T2's catalytic efficiency in degrading both this compound and 2-methylthis compound. [] Structural analyses of human T2 reveal that K+ binds near the CoA binding site and the catalytic site, leading to the stabilization of crucial loops involved in substrate binding and catalysis. [] This interaction underscores the importance of potassium ions for optimal T2 activity.
Q9: How does this compound affect insulin secretion in pancreatic beta cells?
A9: this compound plays a significant role in glucose-stimulated insulin secretion (GSIS). [] In pancreatic beta cells, this compound is synthesized from glucose and leucine in the mitochondria. [] It is then transferred to the cytosol, where it is converted to other short-chain acyl-CoAs, contributing to GSIS. [] Knockdown studies targeting this compound synthetase, a key enzyme in the cytosolic formation of this compound, resulted in partial inhibition of GSIS. [] This finding suggests that the cytosolic pool of this compound is crucial for optimal insulin secretion. []
Q10: What is the role of this compound in proinflammatory macrophage activation?
A10: Research indicates that this compound, derived from fatty acid synthase (FASN) activity, is essential for lipopolysaccharide-induced TLR4-mediated macrophage activation. [] Interestingly, the role of this compound in this context appears to be independent of its function in palmitate synthesis. [] Instead, this compound promotes cholesterol production, which is essential for TLR4 recruitment to lipid rafts and subsequent signal transduction. [] This study highlights a previously unrecognized link between FASN, cholesterol synthesis, and TLR4 signaling in macrophages during inflammation. []
Q11: How is this compound typically measured in biological samples?
A11: this compound levels can be measured using various techniques, including:
Q12: What are some of the key historical milestones in the research of this compound?
A12: Early research on this compound focused on its role in ketone body metabolism and fatty acid oxidation. [, , ] The discovery of this compound thiolase and its ping-pong kinetic mechanism was a major milestone in understanding the regulation of these pathways. [, ] Later, the involvement of this compound in cholesterol and isoprenoid biosynthesis through the mevalonate pathway broadened its metabolic significance. [, , ] More recently, research has uncovered the role of this compound in diverse cellular processes, such as GSIS in pancreatic beta cells [] and proinflammatory macrophage activation. []
Disclaimer and Information on In-Vitro Research Products
Please be aware that all articles and product information presented on BenchChem are intended solely for informational purposes. The products available for purchase on BenchChem are specifically designed for in-vitro studies, which are conducted outside of living organisms. In-vitro studies, derived from the Latin term "in glass," involve experiments performed in controlled laboratory settings using cells or tissues. It is important to note that these products are not categorized as medicines or drugs, and they have not received approval from the FDA for the prevention, treatment, or cure of any medical condition, ailment, or disease. We must emphasize that any form of bodily introduction of these products into humans or animals is strictly prohibited by law. It is essential to adhere to these guidelines to ensure compliance with legal and ethical standards in research and experimentation.