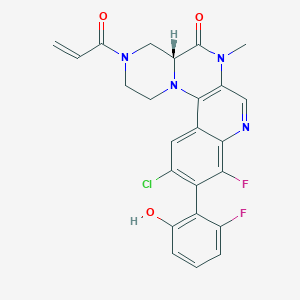
KRAS G12C inhibitor 14
- Click on QUICK INQUIRY to receive a quote from our team of experts.
- With the quality product at a COMPETITIVE price, you can focus more on your research.
Overview
Description
KRAS G12C inhibitor 14 is a small-molecule compound designed to target the KRAS G12C mutation, a common genetic alteration found in various cancers, including non-small cell lung cancer, colorectal cancer, and pancreatic cancer . This compound binds covalently to the KRAS G12C mutant protein, locking it in an inactive state and thereby inhibiting its oncogenic signaling pathways .
Preparation Methods
Synthetic Routes and Reaction Conditions
The synthesis of KRAS G12C inhibitor 14 involves multiple steps, including the formation of key intermediates and the final coupling reaction. The synthetic route typically starts with the preparation of a core scaffold, followed by functional group modifications to introduce the desired substituents . Reaction conditions often involve the use of organic solvents, catalysts, and specific temperature and pressure settings to optimize yield and purity .
Industrial Production Methods
Industrial production of this compound requires scaling up the laboratory synthesis to a larger scale while maintaining the quality and consistency of the product. This involves optimizing reaction conditions, purification processes, and quality control measures to ensure the final product meets regulatory standards .
Chemical Reactions Analysis
Types of Reactions
KRAS G12C inhibitor 14 undergoes various chemical reactions, including:
Covalent Bond Formation: The compound forms a covalent bond with the cysteine residue in the KRAS G12C mutant protein.
Substitution Reactions: Functional group substitutions are common during the synthesis process.
Common Reagents and Conditions
Common reagents used in the synthesis of this compound include organic solvents, catalysts, and specific reactants to introduce functional groups . Reaction conditions such as temperature, pressure, and pH are carefully controlled to optimize the reactions .
Major Products
The major product of the synthesis is this compound, which is obtained after purification and quality control processes .
Scientific Research Applications
KRAS G12C inhibitor 14 has a wide range of scientific research applications, including:
Mechanism of Action
KRAS G12C inhibitor 14 exerts its effects by binding covalently to the cysteine residue in the KRAS G12C mutant protein, locking it in an inactive GDP-bound state . This prevents the activation of downstream signaling pathways, such as the RAF-MEK-ERK and PI3K-AKT pathways, which are involved in cell proliferation and survival . By inhibiting these pathways, this compound effectively suppresses the growth of cancer cells harboring the KRAS G12C mutation .
Comparison with Similar Compounds
KRAS G12C inhibitor 14 is part of a class of compounds designed to target the KRAS G12C mutation. Similar compounds include:
Sotorasib: The first FDA-approved KRAS G12C inhibitor, known for its efficacy in non-small cell lung cancer.
Adagrasib: Another KRAS G12C inhibitor currently being investigated in clinical trials.
143D: A novel KRAS G12C inhibitor with potent antitumor activity in preclinical models.
Compared to these compounds, this compound may offer unique advantages in terms of potency, selectivity, and pharmacokinetic properties .
Properties
Molecular Formula |
C24H19ClF2N4O3 |
---|---|
Molecular Weight |
484.9 g/mol |
IUPAC Name |
(7R)-16-chloro-14-fluoro-15-(2-fluoro-6-hydroxyphenyl)-9-methyl-5-prop-2-enoyl-2,5,9,12-tetrazatetracyclo[8.8.0.02,7.013,18]octadeca-1(10),11,13,15,17-pentaen-8-one |
InChI |
InChI=1S/C24H19ClF2N4O3/c1-3-18(33)30-7-8-31-16(11-30)24(34)29(2)15-10-28-22-12(23(15)31)9-13(25)19(21(22)27)20-14(26)5-4-6-17(20)32/h3-6,9-10,16,32H,1,7-8,11H2,2H3/t16-/m1/s1 |
InChI Key |
NZIBTXKFKUORFB-MRXNPFEDSA-N |
Isomeric SMILES |
CN1C2=C(C3=CC(=C(C(=C3N=C2)F)C4=C(C=CC=C4F)O)Cl)N5CCN(C[C@@H]5C1=O)C(=O)C=C |
Canonical SMILES |
CN1C2=C(C3=CC(=C(C(=C3N=C2)F)C4=C(C=CC=C4F)O)Cl)N5CCN(CC5C1=O)C(=O)C=C |
Origin of Product |
United States |
Disclaimer and Information on In-Vitro Research Products
Please be aware that all articles and product information presented on BenchChem are intended solely for informational purposes. The products available for purchase on BenchChem are specifically designed for in-vitro studies, which are conducted outside of living organisms. In-vitro studies, derived from the Latin term "in glass," involve experiments performed in controlled laboratory settings using cells or tissues. It is important to note that these products are not categorized as medicines or drugs, and they have not received approval from the FDA for the prevention, treatment, or cure of any medical condition, ailment, or disease. We must emphasize that any form of bodily introduction of these products into humans or animals is strictly prohibited by law. It is essential to adhere to these guidelines to ensure compliance with legal and ethical standards in research and experimentation.