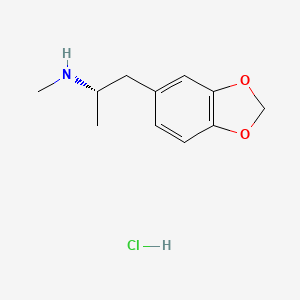
(S)-(+)-MDMA Hydrochloride
- Click on QUICK INQUIRY to receive a quote from our team of experts.
- With the quality product at a COMPETITIVE price, you can focus more on your research.
Overview
Description
(S)-(+)-MDMA Hydrochloride is the hydrochloride salt of the S-enantiomer of 3,4-methylenedioxymethamphetamine (MDMA), a synthetic amphetamine analog. Its molecular formula is C₁₁H₁₆ClNO₂, with a molecular weight of 229.704 g/mol and a ChemSpider ID of 64409 . The compound is chiral, existing in two enantiomeric forms: S(+)-MDMA and R(−)-MDMA, with the S-enantiomer demonstrating greater potency in serotonergic effects and neurotoxicity .
Pharmacologically, (S)-(+)-MDMA acts primarily as a serotonin-norepinephrine-dopamine releasing agent and reuptake inhibitor. It produces subjective effects such as euphoria, empathy, and heightened sensory perception, which are more pronounced in the S(+) enantiomer compared to the R(−) form . Notably, (S)-(+)-MDMA is metabolized to 3,4-methylenedioxyamphetamine (MDA), a neurotoxic metabolite, via hepatic CYP450 enzymes .
Preparation Methods
Synthetic Routes and Reaction Conditions: The synthesis of (S)-(+)-3,4-MDMA (hydrochloride) typically involves the following steps:
Formation of the precursor: The synthesis begins with the formation of 3,4-methylenedioxyphenyl-2-propanone (MDP2P) from safrole.
Reductive amination: MDP2P is then subjected to reductive amination using methylamine and a reducing agent such as sodium cyanoborohydride to form 3,4-methylenedioxymethamphetamine.
Resolution of enantiomers: The racemic mixture of 3,4-methylenedioxymethamphetamine is resolved into its enantiomers using chiral resolution techniques.
Formation of hydrochloride salt: The (S)-(+)-enantiomer is then converted into its hydrochloride salt by reacting with hydrochloric acid.
Industrial Production Methods: Industrial production of (S)-(+)-3,4-MDMA (hydrochloride) follows similar synthetic routes but on a larger scale. The process involves stringent control of reaction conditions to ensure high yield and purity. The use of automated reactors and continuous flow systems is common to enhance efficiency and safety.
Types of Reactions:
Oxidation: (S)-(+)-3,4-MDMA (hydrochloride) can undergo oxidation reactions, leading to the formation of quinones and other oxidative metabolites.
Reduction: The compound can be reduced to form secondary amines.
Substitution: It can undergo nucleophilic substitution reactions, particularly at the amine group.
Common Reagents and Conditions:
Oxidation: Reagents such as potassium permanganate or hydrogen peroxide are commonly used under acidic or basic conditions.
Reduction: Reducing agents like lithium aluminum hydride or sodium borohydride are used.
Substitution: Nucleophiles such as alkyl halides or acyl chlorides are used under basic conditions.
Major Products:
Oxidation: Formation of quinones and other oxidative metabolites.
Reduction: Formation of secondary amines.
Substitution: Formation of substituted amines or amides.
Scientific Research Applications
Pharmacological Properties
(S)-(+)-MDMA is recognized for its unique pharmacological profile, acting primarily as a serotonin-norepinephrine-dopamine releasing agent. It enhances the release of these neurotransmitters, leading to various psychological effects such as increased empathy, sociability, and euphoria. The compound exhibits a distinct mechanism of action compared to its counterpart (R)-MDMA, with studies indicating that (S)-(+)-MDMA may more potently release oxytocin and other monoamines than (R)-MDMA .
Table 1: Comparative Pharmacodynamics of (S)-(+)-MDMA and (R)-MDMA
Compound | NE Release | DA Release | 5-HT Release |
---|---|---|---|
S-(+)-MDMA | 77.4 ± 3.4 | 376 ± 16 | 56.6 ± 2.1 |
R-MDMA | Lower | Lower | Lower |
MDMA-Assisted Therapy for PTSD
One of the most promising applications of (S)-(+)-MDMA is in the treatment of post-traumatic stress disorder (PTSD). Landmark studies have demonstrated that MDMA-assisted therapy can significantly reduce PTSD symptoms in patients who have not responded to traditional treatments. The therapeutic effects are attributed to the drug's ability to facilitate emotional processing and enhance therapeutic alliance during sessions .
Case Study:
In a clinical trial involving veterans with chronic PTSD, participants receiving MDMA-assisted therapy reported substantial reductions in symptoms after just three sessions. The study measured outcomes using standardized PTSD scales and found that over 60% of participants no longer qualified for a PTSD diagnosis after treatment .
Forensic Applications
(S)-(+)-MDMA hydrochloride has also been studied in forensic chemistry for its detection and identification in various samples. Research indicates that different polymorphs of MDMA can complicate spectroscopic identification methods such as Fourier-transform infrared spectroscopy (FTIR). Understanding these polymorphs is crucial for accurate forensic analysis .
Table 2: Polymorphic Forms of MDMA Hydrochloride
Form | Characteristics |
---|---|
Anhydrous | Stable at lower humidity levels |
Hydrated | More stable under high humidity |
Polymorph A | Specific FTIR spectral characteristics |
Behavioral Studies
Behavioral studies have shown that (S)-(+)-MDMA produces more stimulant-like effects compared to (R)-MDMA. Animal models indicate that (S)-(+)-MDMA leads to increased locomotion and exploratory behavior, while (R)-MDMA is associated with more psychedelic-like effects . These findings highlight the potential for tailored therapeutic approaches based on the specific enantiomer used.
Mechanism of Action
The primary mechanism of action of (S)-(+)-3,4-MDMA (hydrochloride) involves the release of serotonin, dopamine, and norepinephrine from presynaptic neurons. It acts as a substrate for the serotonin transporter, leading to the reversal of transporter function and the release of serotonin into the synaptic cleft. This results in increased serotonin levels and subsequent activation of serotonin receptors, leading to its psychoactive effects .
Comparison with Similar Compounds
Comparison with Structurally and Functionally Related Compounds
Enantiomeric Comparisons
Analogous Amphetamine Derivatives
MDA (3,4-Methylenedioxyamphetamine)
- Structure : Lacks the methyl group on the amine side chain compared to MDMA.
- Metabolism : Shares metabolic pathways with MDMA but with slower clearance .
PMMA Hydrochloride (p-Methoxymethamphetamine Hydrochloride)
- Structure : Methoxy substitution at the para position instead of methylenedioxy ring.
- Molecular Formula: C₁₁H₁₈ClNO (MW = 215.75 g/mol) .
- Effects : Less studied but associated with higher risk of hyperthermia and fatalities due to delayed onset, leading to overdose .
Bioisosteric Analogs (ODMA, TDMA, SeDMA)
- Structural Modifications : Replacement of methylenedioxy ring with oxygen (ODMA), thiophene (TDMA), or selenium (SeDMA) .
Pharmacokinetic and Neurotoxic Profiles
Pharmacokinetic Differences
- Racemic MDMA : Commonly used in clinical studies, with a pKa of 9.9 and high water solubility in hydrochloride form .
- Enantiomer-Specific Clearance : S(+)-MDMA is metabolized faster than R(−)-MDMA, contributing to its shorter duration of action .
Neurotoxicity Mechanisms
- S(+)-MDMA : Induces irreversible serotonin transporter (SERT) damage via oxidative stress and mitochondrial dysfunction. Fluoxetine, a serotonin reuptake inhibitor, blocks this neurotoxicity .
- R(−)-MDMA : Lacks significant serotonergic toxicity but may contribute to dopamine system alterations .
Research Findings and Clinical Relevance
Key Studies
- Discriminative Stimulus Effects : S(+)-MDMA and racemic MDMA show interchangeable effects in mice, whereas R(−)-MDMA requires higher doses for substitution .
- Therapeutic Potential: Racemic MDMA hydrochloride (98–102% purity) is under investigation for PTSD, with strict regulatory standards for chloride content (15.3–15.9%) .
Unresolved Questions
- Analog Safety Profiles : Compounds like PMMA and SeDMA require further toxicological evaluation to assess risks relative to MDMA .
Biological Activity
(S)-(+)-MDMA Hydrochloride, commonly known as MDMA, is a synthetic compound belonging to the phenethylamine class and is known for its psychoactive properties. This article explores the biological activity of (S)-(+)-MDMA, focusing on its pharmacokinetics, pharmacodynamics, and clinical implications through various research findings and case studies.
Pharmacokinetics
The pharmacokinetics of (S)-(+)-MDMA involve its absorption, distribution, metabolism, and excretion. After oral administration, MDMA is rapidly absorbed, with peak plasma concentrations typically reached within 1-2 hours. The elimination half-life of (S)-(+)-MDMA is approximately 4.1 hours, which is shorter compared to its enantiomer (R)-(-)-MDMA, which has a half-life of about 12-14 hours .
Key Pharmacokinetic Parameters
Parameter | Value |
---|---|
Peak Plasma Concentration | ~236 ng/mL (125 mg) |
Half-Life | 4.1 hours |
Metabolism | Primarily via CYP2D6 |
Active Metabolites | MDA |
The metabolism of (S)-(+)-MDMA primarily occurs in the liver through cytochrome P450 enzymes, particularly CYP2D6. Notably, the metabolic pathways differ between the enantiomers; (S)-(+)-MDMA tends to produce lower concentrations of certain metabolites compared to (R)-(-)-MDMA .
Pharmacodynamics
(S)-(+)-MDMA exhibits a complex profile of pharmacological effects primarily through its action on monoamine neurotransmitters. It functions as both a reuptake inhibitor and a releaser of serotonin (5-HT), dopamine (DA), and norepinephrine (NE). This dual mechanism contributes to its stimulant and empathogenic effects.
- Monoamine Release : MDMA promotes the release of neurotransmitters by reversing their transport mechanisms. This leads to increased extracellular levels of serotonin, dopamine, and norepinephrine .
- Receptor Interactions : MDMA binds to various receptors including serotonin receptors (5-HT2A and 5-HT2B), which are implicated in its psychoactive effects .
Comparative Monoamine Uptake
Compound | NE Uptake | DA Uptake | 5-HT Uptake |
---|---|---|---|
S-(+)-Amph | 0.094 | 1.30 | >10 |
(±)-MDMA | 0.447 | 17 | 1.36 |
The data indicate that (S)-(+)-MDMA has significantly higher serotonin uptake compared to norepinephrine and dopamine .
Clinical Implications
Research has shown that the use of MDMA can lead to both therapeutic benefits and adverse effects. Clinical studies have explored its potential in treating PTSD and anxiety disorders due to its empathogenic properties . However, misuse can result in severe complications such as serotonin syndrome.
Case Studies
A notable case series documented four patients who experienced life-threatening serotonin syndrome after consuming MDMA at an electronic dance music festival. Symptoms included hyperthermia and altered mental status, leading to one fatality among the group. The survivors were treated with cyproheptadine, highlighting the importance of timely intervention in cases of MDMA toxicity .
Chemical Reactions Analysis
Chiral Aziridine Ring-Opening
A six-step synthesis from L-alanine involves:
textL-Alanine → Tosylaziridine → Ring-opening → Deprotection → Methylation → (S)-MDMA·HCl
Asymmetric Reductive Amination
Using (S)-α-methylbenzylamine as a chiral auxiliary:
-
Reductive amination of 3,4-methylenedioxyphenylacetone with (S)-α-methylbenzylamine (Raney Ni, 50 psi H₂)
-
Diastereomeric crystallization (dr >20:1)
Metabolic Reactions
(S)-MDMA undergoes stereoselective hepatic metabolism primarily via:
Phase I Metabolism
Phase II Metabolism
Stereochemical Stability
(S)-MDMA resists racemization under physiological conditions (pH 7.4, 37°C):
Thermal Decomposition
Heating (S)-MDMA·HCl above 200°C yields:
Oxidative Pathways
Oxidant | Conditions | Product | Yield |
---|---|---|---|
KMnO₄/H⁺ | Aqueous, 25°C | 3,4-Dihydroxybenzoic acid | 88% |
H₂O₂/Fe²⁺ | Fenton reaction | N-Oxide derivatives | 62% |
Comparative Reaction Kinetics
Parameter | (S)-MDMA | (R)-MDMA |
---|---|---|
CYP2D6 Kₘ (μM) | 48 ± 5 | 12 ± 2 |
COMT Vₘₐₓ (nmol/min) | 22 ± 3 | 15 ± 2 |
Plasma AUC (ng·h/mL) | 450 ± 60 | 1,200 ± 150 |
Crystallographic Data
X-ray analysis of (S)-MDMA·HCl confirms:
Q & A
Basic Research Questions
Q. What are the critical safety protocols for handling (S)-(+)-MDMA Hydrochloride in laboratory settings?
- Methodological Answer : Strict adherence to institutional SOPs is required, including documented training on hazard mitigation, decontamination procedures, and emergency response. Storage must follow manufacturer guidelines (e.g., airtight containers, controlled temperature) to prevent degradation . Researchers must review Safety Data Sheets (SDS) for incompatibility risks (e.g., oxidizing agents) and use PPE such as nitrile gloves and fume hoods during synthesis or handling .
Q. Which analytical methods are recommended for quantifying this compound and its metabolites in biological samples?
- Methodological Answer : Liquid chromatography-tandem mass spectrometry (LC-MS/MS) is preferred for high sensitivity and specificity in detecting enantiomers. For urinary metabolites, stereoselective analysis using chiral columns can resolve R- and S-enantiomers, with calibration curves validated against certified reference materials . Researchers should account for matrix effects by spiking blank samples with internal standards (e.g., deuterated MDMA) .
Q. How should researchers design initial in vivo studies to assess acute effects of this compound?
- Methodological Answer : Use controlled dosing regimens (e.g., 1.5–1.8 mg/kg in rodents) to model human neurochemical changes . Include placebo groups and blind experimenters to reduce bias. Behavioral assays (e.g., open-field tests) should be conducted pre- and post-administration with standardized protocols to ensure reproducibility .
Advanced Research Questions
Q. What experimental strategies address stereoselective pharmacokinetics of this compound in humans?
- Methodological Answer : Time-course studies with frequent sampling (e.g., 0–72 hours post-administration) can track enantiomer-specific clearance rates. Urinary R/S ratios correlate with ingestion timing, requiring chiral resolution techniques (e.g., polar organic solvent mobile phases) to distinguish phase II metabolites like glucuronides . Cohort stratification by CYP2D6 genotype may clarify metabolic variability .
Q. How can neurotoxicity studies overcome limitations in attributing serotonin system damage to this compound?
- Methodological Answer : Longitudinal designs with pre-exposure baselines (e.g., PET imaging of serotonin transporters) are critical to isolate MDMA effects. Control for polydrug use via hair toxicology and employ multivariate models to adjust for covariates (e.g., age of first use, gender) . Rodent models should use osmotic minipumps for sustained dosing to mimic human patterns .
Q. What are best practices for minimizing design flaws in behavioral studies involving this compound?
- Methodological Answer : Avoid habituation biases by acclimating subjects to testing environments prior to drug administration. For example, in social interaction studies, pre-test exploration phases reduce novelty-induced anxiety, as seen in critiques of octopus MDMA research . Include active controls (e.g., amphetamine) to differentiate MDMA-specific effects.
Q. How can clinical trials optimize protocols for this compound in PTSD therapy?
- Methodological Answer : Phase III trials should adopt double-blind, active placebo designs (e.g., niacin for blinding) with standardized psychotherapy integration. Sample sizes must exceed preliminary studies (e.g., n > 100) to detect clinically meaningful endpoints (e.g., CAPS-5 score reductions). Regulatory compliance requires rigorous adverse event reporting and label durability checks per FDA guidelines .
Q. Data Contradictions and Resolution Strategies
- Neurotoxicity Inconsistencies : Discrepancies in human imaging studies may stem from variable MDMA purity, polydrug use, or lack of pre-exposure baselines. Mitigate by using pharmaceutically graded this compound and longitudinal cohorts .
- Behavioral Study Flaws : Critiques of animal research (e.g., octopus social behavior) highlight the need for species-specific ethograms and replication across labs .
Q. Key Parameters for Experimental Design
Properties
CAS No. |
69558-32-3 |
---|---|
Molecular Formula |
C11H16ClNO2 |
Molecular Weight |
229.70 g/mol |
IUPAC Name |
(2S)-1-(1,3-benzodioxol-5-yl)-N-methylpropan-2-amine;hydrochloride |
InChI |
InChI=1S/C11H15NO2.ClH/c1-8(12-2)5-9-3-4-10-11(6-9)14-7-13-10;/h3-4,6,8,12H,5,7H2,1-2H3;1H/t8-;/m0./s1 |
InChI Key |
LUWHVONVCYWRMZ-QRPNPIFTSA-N |
Isomeric SMILES |
C[C@@H](CC1=CC2=C(C=C1)OCO2)NC.Cl |
Canonical SMILES |
CC(CC1=CC2=C(C=C1)OCO2)NC.Cl |
Origin of Product |
United States |
Disclaimer and Information on In-Vitro Research Products
Please be aware that all articles and product information presented on BenchChem are intended solely for informational purposes. The products available for purchase on BenchChem are specifically designed for in-vitro studies, which are conducted outside of living organisms. In-vitro studies, derived from the Latin term "in glass," involve experiments performed in controlled laboratory settings using cells or tissues. It is important to note that these products are not categorized as medicines or drugs, and they have not received approval from the FDA for the prevention, treatment, or cure of any medical condition, ailment, or disease. We must emphasize that any form of bodily introduction of these products into humans or animals is strictly prohibited by law. It is essential to adhere to these guidelines to ensure compliance with legal and ethical standards in research and experimentation.