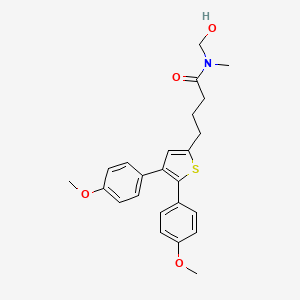
N-Hydroxy-N-methyl-4-(2,3-bis(4-methoxyphenyl)thiophen-5-yl)butanamide
- Click on QUICK INQUIRY to receive a quote from our team of experts.
- With the quality product at a COMPETITIVE price, you can focus more on your research.
Overview
Description
N-Hydroxy-N-methyl-4-(2,3-bis(4-methoxyphenyl)thiophen-5-yl)butanamide is a synthetic compound featuring a thiophene core substituted with two 4-methoxyphenyl groups at the 2- and 3-positions. The butanamide side chain includes an N-hydroxy-N-methyl moiety, which classifies it as a hydroxamic acid derivative. The compound’s synthesis likely involves coupling reactions to construct the thiophene ring, followed by amidation steps to introduce the hydroxamic acid group. While direct spectral or crystallographic data for this compound are absent, its structural analogs (e.g., methoxyphenyl-substituted heterocycles) suggest characteristic spectral signatures, such as methoxy proton resonances at δ ~3.8–3.9 ppm in $ ^1H $-NMR and C=O stretches near 1660–1680 cm$ ^{-1} $ in IR spectra .
Preparation Methods
The synthesis of S 19812 involves a multi-step processThe reaction conditions typically involve the use of organic solvents, catalysts, and controlled temperatures to ensure the desired product yield and purity . Industrial production methods for S 19812 would likely involve scaling up these synthetic routes while optimizing reaction conditions to maximize efficiency and minimize costs.
Chemical Reactions Analysis
S 19812 undergoes various chemical reactions, including oxidation, reduction, and substitution. Common reagents used in these reactions include oxidizing agents like hydrogen peroxide, reducing agents such as sodium borohydride, and nucleophiles for substitution reactions. The major products formed from these reactions depend on the specific conditions and reagents used. For example, oxidation of S 19812 can lead to the formation of sulfoxides or sulfones, while reduction can yield the corresponding alcohols or amines .
Scientific Research Applications
S 19812 has been extensively studied for its potential applications in various fields of scientific research. In chemistry, it serves as a model compound for studying dual inhibition mechanisms of cyclooxygenase and lipoxygenase pathways. In biology and medicine, S 19812 has shown significant anti-inflammatory and analgesic properties, making it a potential candidate for the treatment of conditions such as osteoarthritis and rheumatoid arthritis . Additionally, its excellent gastric tolerance makes it a promising alternative to traditional non-steroidal anti-inflammatory drugs (NSAIDs) .
Mechanism of Action
The mechanism of action of S 19812 involves the inhibition of both cyclooxygenase and lipoxygenase enzymes. By blocking these pathways, S 19812 reduces the production of pro-inflammatory mediators such as prostaglandins and leukotrienes. This dual inhibition results in effective pain relief and anti-inflammatory effects. The molecular targets of S 19812 include the active sites of cyclooxygenase and lipoxygenase enzymes, where it binds and prevents the conversion of arachidonic acid to its inflammatory metabolites .
Comparison with Similar Compounds
Comparison with Similar Compounds
Structural Analogues with Bis(4-methoxyphenyl) Substitutents
2.1.1. 2,3-Bis(4-methoxyphenyl)quinoxaline ()
- Core Structure: Quinoxaline (benzene fused to a pyrazine ring) vs. thiophene (5-membered sulfur-containing ring).
- Functional Groups : Both compounds share bis(4-methoxyphenyl) substituents but differ in the heterocyclic core.
- Physical Properties: The quinoxaline derivative has a melting point of 148–150°C, suggesting high crystallinity due to planar aromaticity. The thiophene-based target compound may exhibit lower melting points due to reduced rigidity.
- Spectral Data: $ ^1H $-NMR: Methoxy protons at δ 3.84 ppm (quinoxaline) vs. expected δ ~3.8–3.9 ppm (target compound). IR: Absence of C=O in quinoxaline vs. prominent amide C=O stretch (~1660 cm$ ^{-1}$) in the target .
2,3-Bis(4-methoxyphenyl)-5-phenyltetrazolium Chloride ()
- Core Structure : Tetrazole (5-membered ring with four nitrogen atoms) vs. thiophene.
- Functional Groups : Tetrazolium chloride includes a charged tetrazole ring, contrasting with the neutral thiophene and hydroxamic acid groups in the target.
- Molecular Weight : 396.87 g/mol (tetrazolium) vs. ~455 g/mol (estimated for the target compound).
- Bioactivity : Tetrazolium salts are often used as redox indicators, whereas hydroxamic acids are associated with enzyme inhibition (e.g., histone deacetylases) .
Amide and Thioamide Derivatives ()
5-(4-(4-X-phenylsulfonyl)phenyl)-4-(2,4-difluorophenyl)-2H-1,2,4-triazole-3(4H)-thiones [7–9]
- Core Structure : 1,2,4-Triazole vs. thiophene.
- Functional Groups : Thione (C=S) vs. hydroxamic acid (N–OH).
- Tautomerism : Triazole-thione tautomerism is observed in [7–9], while the thiophene core lacks such behavior.
- Spectral Data :
S-Alkylated 1,2,4-Triazoles [10–15]
- Side Chains : Alkylated thioether groups vs. butanamide chain.
- Electronic Effects : Sulfonyl groups in [10–15] enhance electrophilicity, whereas methoxyphenyl groups in the target compound donate electrons via resonance.
Pharmacopeial Amides ()
Compounds m, n, and o in are structurally complex amides with tetrahydropyrimidinyl and phenoxyacetamido groups.
- Comparison : These compounds lack the thiophene core and methoxyphenyl substituents but share amide functionalities. Their stereochemical complexity (multiple chiral centers) contrasts with the planar thiophene system in the target compound .
Data Tables
Table 1: Key Structural and Physical Comparisons
Research Findings and Implications
- Bioactivity Potential: Hydroxamic acid derivatives are known for enzyme inhibition, suggesting the target compound could interact with metalloenzymes, though this requires experimental validation.
- Synthetic Challenges : The absence of direct synthesis data for the target compound highlights gaps in literature; methods from (e.g., cyclization) may need adaptation for thiophene systems .
Biological Activity
N-Hydroxy-N-methyl-4-(2,3-bis(4-methoxyphenyl)thiophen-5-yl)butanamide, also known as S 19812, is a compound of significant interest due to its dual inhibitory effects on cyclooxygenase (COX) and lipoxygenase (LOX) pathways. This article explores its biological activity, synthesis, and potential therapeutic applications.
Chemical Structure and Properties
The compound has the following chemical formula:
- Molecular Formula : C₂₀H₂₃N₁O₂S
- Molecular Weight : 355.47 g/mol
The structure features a butanamide backbone with a hydroxyl group and a complex thiophene moiety substituted with methoxyphenyl groups. This configuration is crucial for its biological activity.
S 19812 exhibits its biological activity primarily through the inhibition of two key enzymes involved in inflammatory processes:
- Cyclooxygenase (COX) : Enzymes that convert arachidonic acid into prostaglandins, which are mediators of inflammation and pain.
- Lipoxygenase (LOX) : Enzymes that convert arachidonic acid into leukotrienes, which are also involved in inflammatory responses.
Inhibition Data
- IC50 Values :
- PGE2 (Prostaglandin E2): 0.10 µmol/L
- LTB4 (Leukotriene B4): 0.07 µmol/L
- ED50 Values (in vivo):
- PGE2: 13.1 mg/kg
- LTB4: 20.8 mg/kg
These values indicate that S 19812 is a potent inhibitor of both COX and LOX pathways, making it a promising candidate for treating inflammatory conditions such as osteoarthritis .
Therapeutic Potential
The dual inhibition mechanism suggests that S 19812 could be effective in managing pain and inflammation associated with various conditions, particularly osteoarthritis. Its ability to target both COX and LOX pathways may provide a more comprehensive approach to inflammation management compared to traditional NSAIDs, which typically target only COX enzymes.
Case Studies and Research Findings
Several studies have investigated the efficacy of S 19812:
- In Vitro Studies : Research demonstrated that S 19812 effectively inhibited prostaglandin and leukotriene production in rat polymorphonuclear neutrophils (PMNs), confirming its potential role in modulating inflammatory responses .
- In Vivo Studies : Animal models showed significant reductions in inflammatory markers when treated with S 19812, supporting its therapeutic potential for pain relief .
Summary of Findings
Parameter | Value |
---|---|
Molecular Formula | C₂₀H₂₃N₁O₂S |
Molecular Weight | 355.47 g/mol |
IC50 (PGE2) | 0.10 µmol/L |
IC50 (LTB4) | 0.07 µmol/L |
ED50 (PGE2) | 13.1 mg/kg |
ED50 (LTB4) | 20.8 mg/kg |
Q & A
Q. Basic: What are the standard synthetic routes for synthesizing N-Hydroxy-N-methyl-4-(2,3-bis(4-methoxyphenyl)thiophen-5-yl)butanamide?
The compound is synthesized via multi-step routes involving:
- Thiophene core formation : Suzuki-Miyaura coupling of 4-methoxyphenyl boronic acids with brominated thiophene precursors under palladium catalysis.
- Side-chain introduction : Amidation or esterification reactions to attach the N-hydroxy-N-methylbutanamide moiety, often using coupling agents like EDCI/HOBt.
- Protection/deprotection strategies : Hydroxyl groups may require protection (e.g., acetyl) during synthesis to prevent side reactions .
Characterization : Intermediate purity is verified via TLC, and final compounds are confirmed by 1H/13C NMR, IR, and high-resolution mass spectrometry .
Q. Advanced: How can crystallization challenges (e.g., twinning, disorder) be addressed during structural elucidation?
- Twinning : Use SHELXL’s
TWIN
command with BASF parameter refinement. For pseudo-merohedral twinning, HKLF 5 format data is recommended . - Disordered regions : Apply
PART
,SUMP
, andEADP
constraints to model split positions. Anisotropic displacement parameters (ADPs) should be refined for non-H atoms . - Validation : Cross-validate refinement with R-value convergence checks and residual density maps (<±0.5 eÅ−3) .
Q. Basic: What spectroscopic and chromatographic methods confirm the compound’s structural identity and purity?
- NMR : 1H NMR (400 MHz, DMSO-d6) identifies aromatic protons (δ 6.8–7.5 ppm) and N-methyl groups (δ 2.8–3.2 ppm). 13C NMR confirms carbonyl (δ ~170 ppm) and thiophene carbons .
- HPLC : Reverse-phase chromatography (e.g., Newcrom R1 column, acetonitrile/water gradient) assesses purity (>95%) .
- Mass spectrometry : ESI-HRMS validates molecular ion peaks (e.g., [M+H]+) with <5 ppm error .
Q. Advanced: How can researchers resolve contradictions between spectroscopic data and crystallographic models?
- Density vs. NMR discrepancies : Check for dynamic disorder (e.g., rotating methoxy groups) using variable-temperature crystallography.
- Torsional mismatches : Compare DFT-optimized geometries (e.g., Gaussian 16) with crystallographic torsion angles .
- Validation tools : Use PLATON’s ADDSYM to detect missed symmetry and check Rint for data quality .
Q. Basic: What in vitro assays are suitable for initial biological activity screening?
- Anticancer : MTT assays against cancer cell lines (e.g., HeLa, MCF-7) at 1–100 μM concentrations .
- Antimicrobial : Broth microdilution (MIC determination) for Gram-positive/negative bacteria and fungi .
- Cytotoxicity : Parallel testing on non-cancerous cells (e.g., HEK293) to assess selectivity .
Q. Advanced: What strategies elucidate the mechanism of action for this compound?
- Enzyme inhibition : Screen against target panels (e.g., kinases, HDACs) using fluorescence polarization or calorimetry .
- Molecular docking : AutoDock Vina or Schrödinger Suite to predict binding poses in homology-modeled targets (e.g., MDMX) .
- SAR studies : Synthesize analogs with modified methoxy or thiophene groups to identify pharmacophores .
Q. Basic: How is compound stability assessed under varying storage conditions?
- Thermal stability : TGA/DSC analysis (25–300°C, 10°C/min) to determine decomposition points .
- Hydrolytic stability : Incubate in PBS (pH 7.4/4.0) at 37°C for 48h; monitor degradation via HPLC .
- Light sensitivity : Expose to UV (254 nm) and track photodegradation products .
Q. Advanced: How can synthetic yields be optimized for large-scale preparation?
- DOE approaches : Use Taguchi methods to optimize reaction parameters (e.g., solvent, temperature, catalyst loading) .
- Flow chemistry : Continuous processing for exothermic steps (e.g., thiophene coupling) to improve reproducibility .
- Catalyst recycling : Immobilize Pd catalysts on silica or magnetic nanoparticles to reduce costs .
Q. Basic: What computational tools predict the compound’s physicochemical properties?
- LogP : Use MarvinSketch or ACD/Labs with atom-based contribution methods .
- pKa : SPARC or ChemAxon for estimating ionization states of the hydroxyamide group .
- Solubility : COSMO-RS or QSPR models trained on PubChem datasets .
Q. Advanced: How can molecular dynamics simulations improve target interaction studies?
Properties
CAS No. |
181308-68-9 |
---|---|
Molecular Formula |
C24H27NO4S |
Molecular Weight |
425.5 g/mol |
IUPAC Name |
4-[4,5-bis(4-methoxyphenyl)thiophen-2-yl]-N-(hydroxymethyl)-N-methylbutanamide |
InChI |
InChI=1S/C24H27NO4S/c1-25(16-26)23(27)6-4-5-21-15-22(17-7-11-19(28-2)12-8-17)24(30-21)18-9-13-20(29-3)14-10-18/h7-15,26H,4-6,16H2,1-3H3 |
InChI Key |
MCRHMCUBEXNPLM-UHFFFAOYSA-N |
Canonical SMILES |
CN(CO)C(=O)CCCC1=CC(=C(S1)C2=CC=C(C=C2)OC)C3=CC=C(C=C3)OC |
Origin of Product |
United States |
Disclaimer and Information on In-Vitro Research Products
Please be aware that all articles and product information presented on BenchChem are intended solely for informational purposes. The products available for purchase on BenchChem are specifically designed for in-vitro studies, which are conducted outside of living organisms. In-vitro studies, derived from the Latin term "in glass," involve experiments performed in controlled laboratory settings using cells or tissues. It is important to note that these products are not categorized as medicines or drugs, and they have not received approval from the FDA for the prevention, treatment, or cure of any medical condition, ailment, or disease. We must emphasize that any form of bodily introduction of these products into humans or animals is strictly prohibited by law. It is essential to adhere to these guidelines to ensure compliance with legal and ethical standards in research and experimentation.