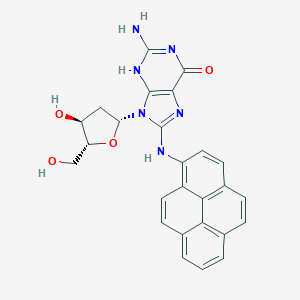
N-(Deoxyguanosin-8-yl)-1-aminopyrene
Overview
Description
N-(Deoxyguanosin-8-yl)-1-aminopyrene (dGAP) is a major DNA adduct formed through the metabolic activation of 1-nitropyrene (1-NP), a potent environmental mutagen and carcinogen commonly found in diesel exhaust and air particulate matter . 1-NP undergoes nitroreduction via mammalian enzymes like xanthine oxidase, producing reactive intermediates such as N-hydroxy-1-aminopyrene, which covalently binds to the C8 position of deoxyguanosine in DNA . This adduct has been extensively characterized in bacterial (e.g., Escherichia coli, Salmonella typhimurium) and mammalian systems (e.g., rat liver, Chinese hamster ovary cells), where it induces frameshift mutations, base substitutions, and deletions . Its mutagenicity is strongly influenced by local DNA sequence context, repair mechanisms, and SOS response activation .
Preparation Methods
Synthetic Routes and Reaction Conditions: The synthesis of N-(Deoxyguanosin-8-yl)-1-aminopyrene typically involves the reaction of 1-aminopyrene with deoxyguanosine. This reaction can be facilitated by using an activated form of 1-aminopyrene, such as N-acetoxy-1-aminopyrene, which reacts with the guanine base in DNA to form the adduct .
Industrial Production Methods: Industrial production methods for this compound are not well-documented, as it is primarily synthesized for research purposes rather than commercial production. The synthesis is usually carried out in specialized laboratories equipped to handle carcinogenic substances.
Chemical Reactions Analysis
Types of Reactions: N-(Deoxyguanosin-8-yl)-1-aminopyrene can undergo various chemical reactions, including:
Oxidation: This reaction can lead to the formation of oxidized derivatives of the compound.
Reduction: Reduction reactions can modify the aminopyrene moiety.
Substitution: Substitution reactions can occur at the amino group or other reactive sites on the molecule.
Common Reagents and Conditions:
Oxidation: Common oxidizing agents include hydrogen peroxide and potassium permanganate.
Reduction: Reducing agents such as sodium borohydride or lithium aluminum hydride can be used.
Substitution: Conditions for substitution reactions vary depending on the desired product but often involve nucleophilic or electrophilic reagents.
Major Products: The major products formed from these reactions depend on the specific conditions and reagents used. For example, oxidation can lead to the formation of hydroxylated derivatives, while reduction can yield deaminated products.
Scientific Research Applications
Mutagenicity Studies
C8-AP-dG has been shown to induce various mutations, including frameshifts and base substitutions, which are critical in understanding its role in carcinogenesis.
- Experimental Findings : In studies involving Escherichia coli and simian kidney (COS-7) cells, C8-AP-dG was found to induce mutations predominantly through frameshift mechanisms. The mutational signature varies between organisms, with notable differences in the types of mutations observed in bacterial systems compared to mammalian cells .
- Comparison with Other Adducts : When compared to other DNA adducts such as those formed by 2-aminofluorene, C8-AP-dG exhibited intermediate toxicity levels. The study indicated that while 2-aminofluorene adducts were more toxic, C8-AP-dG still significantly affected cell viability and mutation rates .
Structural Analysis
Understanding the structural properties of C8-AP-dG is crucial for elucidating its mutagenic mechanisms.
- Spectroscopic Studies : Techniques such as NMR spectroscopy have been employed to analyze the solution structure of C8-AP-dG in DNA duplexes. These studies reveal that the aminopyrene moiety can intercalate into the DNA helix, affecting replication fidelity and stability .
- Conformational Dynamics : Research indicates that C8-AP-dG adopts a syn conformation within the DNA helix, leading to potential replication fork stalling during DNA synthesis. This structural insight is vital for understanding how such adducts can lead to mutations during cell division .
Implications in Cancer Research
The formation of C8-AP-dG is linked to the carcinogenic effects of 1-nitropyrene, making it a focal point in cancer research.
- Tumorigenicity Studies : Animal studies have demonstrated that exposure to 1-nitropyrene leads to the formation of this adduct in various tissues, including liver and lung tissues, which are critical sites for tumor development .
- Mutational Hotspots : The presence of C8-AP-dG at specific loci, such as codon 273 of the p53 tumor suppressor gene, highlights its role in initiating mutations that can lead to cancer. This correlation underscores the importance of studying such adducts in understanding cancer etiology .
Potential Therapeutic Applications
While primarily studied for its mutagenic properties, there is emerging interest in exploring the therapeutic potential of compounds related to C8-AP-dG.
- Targeting DNA Repair Mechanisms : Understanding how cells repair damage caused by C8-AP-dG may lead to novel therapeutic strategies aimed at enhancing DNA repair pathways or targeting cancer cells with specific vulnerabilities related to these adducts .
Data Tables
Case Studies
- Mutagenesis in E. coli : A study demonstrated site-specific mutagenesis induced by C8-AP-dG, revealing a predominance of frameshift mutations when introduced into a dinucleotide repeat sequence .
- DNA Repair Mechanisms : Research has focused on how cells respond to bulky DNA adducts like C8-AP-dG, providing insights into potential therapeutic targets for enhancing repair processes or selectively targeting cancer cells with compromised repair mechanisms .
Mechanism of Action
The mechanism by which N-(Deoxyguanosin-8-yl)-1-aminopyrene exerts its effects involves the formation of a covalent bond between the aminopyrene moiety and the guanine base in DNA. This adduct can cause distortions in the DNA helix, leading to errors during DNA replication and transcription. The primary molecular targets are the DNA repair enzymes that recognize and remove these adducts to prevent mutations .
Comparison with Similar Compounds
Structural and Metabolic Comparisons
Key Insights :
- Positional Specificity: Unlike N2-BPDE-dG, which binds guanine’s exocyclic amino group, dGAP’s C8 linkage induces base displacement and intercalation of the pyrene ring, distorting the DNA helix .
- Metabolic Activation : dGAP forms via nitroreduction , whereas BPDE-dG requires oxidative activation to diolepoxides .
Mutagenic Profiles
Key Insights :
- Frameshift Dominance : dGAP preferentially induces deletions in repetitive sequences (e.g., CGCG sites), whereas BPDE-dG causes targeted transversions .
- SOS Dependence: SOS induction amplifies dGAP’s mutagenicity by 3–5× in E.
- Repair Resistance : dGAC’s bulky, intercalated structure hinders nucleotide excision repair (NER), unlike more accessible N2 adducts .
Conformational and Biophysical Properties
Property | dGAP | N2-BPDE-dG | AF-dG |
---|---|---|---|
DNA Helix Distortion | Base displacement + intercalation | Minor groove distortion | Major groove stacking |
Glycosidic Torsion | Syn conformation | Anti conformation | Syn conformation |
Thermal Stability | Reduced duplex stability | Moderate destabilization | Severe destabilization |
Key Insights :
- Intercalation : dGAP’s pyrene ring intercalates between adjacent base pairs, displacing the modified guanine into the major groove .
- Syn vs. Anti Conformation : The syn glycosidic torsion in dGAC contrasts with the anti conformation of BPDE-dG, altering polymerase bypass efficiency .
Research Findings and Implications
- In Vivo Relevance: dGAP is a major adduct in rodents exposed to 1-NP but a minor adduct in rabbits, highlighting species-specific metabolic differences .
- Environmental Impact: 1-NP’s prevalence in PM2.5 underscores dGAP’s role in pollution-related carcinogenesis .
- Therapeutic Targets : Y-family polymerases (e.g., Pol κ, Dpo4) inefficiently bypass dGAC, suggesting strategies to exploit replication stress .
Biological Activity
N-(Deoxyguanosin-8-yl)-1-aminopyrene (C8-AP-dG) is a significant DNA adduct formed from the interaction of 1-nitropyrene with DNA. This compound has garnered attention due to its mutagenic properties, which are critical for understanding its role in carcinogenesis. This article explores the biological activity of C8-AP-dG, focusing on its mutagenicity, mechanisms of action, and implications in cancer research.
Overview of this compound
C8-AP-dG is a bulky DNA adduct that results from the metabolic activation of 1-nitropyrene, a polycyclic aromatic hydrocarbon (PAH) commonly found in environmental pollutants. The formation of this adduct is a crucial step in the pathway leading to DNA damage and subsequent mutations, which can contribute to cancer development.
Mutational Spectrum
Research indicates that C8-AP-dG induces a variety of mutations in DNA. The mutational spectrum is influenced by the surrounding base sequence, leading to different types of mutations depending on the context. For instance, studies have shown that C8-AP-dG primarily causes frameshift mutations, including one-base deletions and insertions, particularly in repetitive sequences like CpG sites .
Table 1: Types of Mutations Induced by C8-AP-dG
Mutation Type | Description | Frequency |
---|---|---|
Frameshift | One-base deletions and insertions | Predominant |
Base Substitutions | G→C transversions | 1–2% |
Other Mutations | Context-dependent variations | Variable |
Comparative Toxicity
In comparative studies with other DNA adducts such as those formed by 2-aminofluorene (AF) and N-acetyl-2-aminofluorene (AAF), C8-AP-dG exhibited intermediate toxicity. The survival rate of progeny cells post-exposure to these adducts was significantly lower for AAF compared to C8-AP-dG and AF, indicating that while C8-AP-dG is mutagenic, it may not be as lethal as some other adducts .
In Vitro Studies
In vitro experiments using simian kidney (COS-7) cells have demonstrated that the presence of C8-AP-dG leads to a notable reduction in cell viability. The study indicated that translesion synthesis predominantly occurs through error-free pathways, but low levels of mutations were still observed .
A specific focus on the mutational outcomes revealed that when C8-AP-dG was introduced into a dinucleotide repeat sequence in Escherichia coli, it resulted in significant frameshift mutations, underscoring its potential as a potent mutagen .
In Vivo Studies
In vivo studies involving female rats treated with 1-nitropyrene showed that C8-AP-dG was a major DNA adduct formed during metabolism. The mutational analysis revealed that this adduct contributes to the development of tumors, particularly in lung tissues where similar nitroaromatic compounds have been implicated in carcinogenesis .
Implications for Cancer Research
The biological activity of this compound highlights its role as a significant contributor to DNA damage and mutation. Understanding its mechanisms can aid in developing preventive strategies against cancers associated with environmental exposures to nitroaromatic compounds. Furthermore, this knowledge can inform risk assessments related to pollutants and guide future research into therapeutic interventions targeting DNA repair mechanisms.
Q & A
Basic Research Questions
Q. How is N-(deoxyguanosin-8-yl)-1-aminopyrene (dGAP) formed in DNA, and what methodologies are used to detect it?
- Formation : dGAP arises from metabolic activation of 1-nitropyrene (1-NP) via nitroreduction to N-hydroxy-1-aminopyrene, which generates a reactive nitrenium ion. This ion binds covalently to the C8 position of deoxyguanosine in DNA, forming a bulky adduct .
- Detection :
- Liquid chromatography-tandem mass spectrometry (LC-MS/MS) with electrospray ionization (ESI) provides high sensitivity for quantifying dGAP in biological samples .
- 32P-postlabeling is used to detect low levels of adducts in tissues like rat liver and mouse lung .
Q. What experimental models are used to study dGAP-induced mutagenesis, and how are they designed?
- Bacterial systems : Escherichia coli and Salmonella typhimurium with SOS response induction (e.g., umuDC operon) are employed to assess frameshift mutations (e.g., −2 or +1 deletions/insertions) in repetitive sequences like 5'-CGCGCG-3' .
- Mammalian systems : Simian kidney (COS-7) and human embryonic kidney (293T) cells transfected with site-specific adduct-containing plasmids are used to evaluate mutation spectra (e.g., G→T transversions) in tumor suppressor gene contexts (e.g., p53 codon 273) .
- Key variables : SOS induction, mismatch repair deficiency (mutS/mutL), and proofreading-deficient (dnaQ) strains modulate mutation frequency .
Advanced Research Questions
Q. How does the structural conformation of dGAP influence its mutagenic outcomes?
- NMR and computational studies reveal that dG adopts a syn glycosidic torsion angle, causing the aminopyrene ring to intercalate into the DNA helix. This disrupts Watson-Crick base pairing and stabilizes a base-displaced conformation, promoting polymerase stalling and frameshift mutations .
- Sequence context : In a 5'-CGC-3' sequence, dGAP induces −2 deletions via slipped misalignment, while in non-repetitive sequences (e.g., 5'-GTG-3'), G→T transversions dominate due to error-prone bypass by Y-family polymerases (e.g., Pol κ) .
Q. How do researchers reconcile contradictions in mutation patterns between bacterial and mammalian systems?
- Bacterial systems : SOS-dependent translesion synthesis (TLS) by Pol V predominates, favoring frameshifts (e.g., CpG deletions) in repetitive sequences .
- Mammalian systems : Error-prone TLS by Pol η/ι/κ and error-free bypass by Pol ζ lead to base substitutions (e.g., G→T) in non-repetitive contexts. Methylation of 5'-cytosine (5-MeC) adjacent to dGAP enhances G→A transitions by altering polymerase fidelity .
- Validation : Cross-species comparisons using identical DNA sequences (e.g., 5'-CGCGCG-3') highlight repair pathway differences .
Q. What role do epigenetic modifications (e.g., DNA methylation) play in dGAP mutagenesis?
- 5-Methylcytosine (5-MeC) at the 5'-position of dGAP increases mutation frequency by 50–100% in mammalian cells. This is attributed to enhanced adduct stabilization and polymerase misincorporation (e.g., G→A transitions) due to altered base stacking .
- Methodology : Site-specific incorporation of 5-MeC via phosphoramidite chemistry in oligonucleotides, followed by transfection into repair-proficient/defective cell lines .
Q. How do Y-family DNA polymerases bypass dGAP lesions, and what determines error-prone vs. error-free replication?
- Structural determinants : The bulky aminopyrene moiety in dGAP induces a "bulge" or "loop-out" conformation, which Pol η accommodates via its open active site, enabling error-prone bypass. In contrast, Pol ι’s rigid active site increases fidelity .
- Kinetic assays : Pre-steady-state kinetic analysis (e.g., kpol and Kd) reveals that Pol κ exhibits higher efficiency for dCTP incorporation opposite dGAP, reducing mutagenesis .
Q. What repair pathways mitigate dGAP-induced genomic damage, and how efficient are they?
- Nucleotide excision repair (NER) : Limited efficiency due to the adduct’s intrahelical positioning. In vitro assays with human cell extracts show poor recognition by XPC-RAD23B .
- Mismatch repair (MMR) : Corrects ~50% of frameshifts in E. coli but is less effective in mammalian systems. Methyl-directed MMR (mutHLS) reduces mutation frequency by 30–70% .
- Proofreading : The 3'→5' exonuclease activity of DNA Pol III removes misaligned intermediates, decreasing frameshifts by 5–7-fold in dnaQ mutants .
Q. Methodological Challenges and Data Interpretation
Q. How do researchers address discrepancies in adduct quantification between in vitro and in vivo models?
- In vitro : Artificial metabolic activation (e.g., nitroreductase + NADPH) may overestimate adduct levels. Use isotopically labeled dGAP (e.g., <sup>15</sup>N/<sup>13</sup>C) as an internal standard .
- In vivo : Tissue-specific differences (e.g., higher adducts in rat mammary gland vs. liver) require normalization to DNA content and correction for hydrolysis artifacts .
Q. What computational tools predict dGAP’s mutagenic potential based on its conformation?
- Molecular dynamics (MD) simulations : Energy-minimized models (AMBER/CHARMM force fields) correlate adduct flexibility with mutation hotspots. For example, intercalated conformations predict frameshifts, while minor-groove adducts favor base substitutions .
- Docking studies : Predict polymerase-adduct interactions (e.g., Pol η’s "bypass tunnel" accommodating dGAP) to prioritize experimental validation .
Q. Tables
Table 1. Mutation Spectra of dGAP Across Model Systems
Table 2. Key Structural Studies on dGAP
Properties
IUPAC Name |
2-amino-9-[(2R,4S,5R)-4-hydroxy-5-(hydroxymethyl)oxolan-2-yl]-8-(pyren-1-ylamino)-1H-purin-6-one | |
---|---|---|
Source | PubChem | |
URL | https://pubchem.ncbi.nlm.nih.gov | |
Description | Data deposited in or computed by PubChem | |
InChI |
InChI=1S/C26H22N6O4/c27-25-30-23-22(24(35)31-25)29-26(32(23)19-10-17(34)18(11-33)36-19)28-16-9-7-14-5-4-12-2-1-3-13-6-8-15(16)21(14)20(12)13/h1-9,17-19,33-34H,10-11H2,(H,28,29)(H3,27,30,31,35)/t17-,18+,19+/m0/s1 | |
Source | PubChem | |
URL | https://pubchem.ncbi.nlm.nih.gov | |
Description | Data deposited in or computed by PubChem | |
InChI Key |
QBMJHUSBJZIUSS-IPMKNSEASA-N | |
Source | PubChem | |
URL | https://pubchem.ncbi.nlm.nih.gov | |
Description | Data deposited in or computed by PubChem | |
Canonical SMILES |
C1C(C(OC1N2C3=C(C(=O)NC(=N3)N)N=C2NC4=C5C=CC6=CC=CC7=C6C5=C(C=C7)C=C4)CO)O | |
Source | PubChem | |
URL | https://pubchem.ncbi.nlm.nih.gov | |
Description | Data deposited in or computed by PubChem | |
Isomeric SMILES |
C1[C@@H]([C@H](O[C@H]1N2C3=C(C(=O)NC(=N3)N)N=C2NC4=C5C=CC6=CC=CC7=C6C5=C(C=C7)C=C4)CO)O | |
Source | PubChem | |
URL | https://pubchem.ncbi.nlm.nih.gov | |
Description | Data deposited in or computed by PubChem | |
Molecular Formula |
C26H22N6O4 | |
Source | PubChem | |
URL | https://pubchem.ncbi.nlm.nih.gov | |
Description | Data deposited in or computed by PubChem | |
DSSTOX Substance ID |
DTXSID201006568 | |
Record name | 9-(2-Deoxypentofuranosyl)-2-imino-8-[(pyren-1-yl)imino]-3,7,8,9-tetrahydro-2H-purin-6-ol | |
Source | EPA DSSTox | |
URL | https://comptox.epa.gov/dashboard/DTXSID201006568 | |
Description | DSSTox provides a high quality public chemistry resource for supporting improved predictive toxicology. | |
Molecular Weight |
482.5 g/mol | |
Source | PubChem | |
URL | https://pubchem.ncbi.nlm.nih.gov | |
Description | Data deposited in or computed by PubChem | |
CAS No. |
85989-43-1 | |
Record name | N-(Deoxyguanosin-8-yl)-1-aminopyrene | |
Source | ChemIDplus | |
URL | https://pubchem.ncbi.nlm.nih.gov/substance/?source=chemidplus&sourceid=0085989431 | |
Description | ChemIDplus is a free, web search system that provides access to the structure and nomenclature authority files used for the identification of chemical substances cited in National Library of Medicine (NLM) databases, including the TOXNET system. | |
Record name | 9-(2-Deoxypentofuranosyl)-2-imino-8-[(pyren-1-yl)imino]-3,7,8,9-tetrahydro-2H-purin-6-ol | |
Source | EPA DSSTox | |
URL | https://comptox.epa.gov/dashboard/DTXSID201006568 | |
Description | DSSTox provides a high quality public chemistry resource for supporting improved predictive toxicology. | |
Retrosynthesis Analysis
AI-Powered Synthesis Planning: Our tool employs the Template_relevance Pistachio, Template_relevance Bkms_metabolic, Template_relevance Pistachio_ringbreaker, Template_relevance Reaxys, Template_relevance Reaxys_biocatalysis model, leveraging a vast database of chemical reactions to predict feasible synthetic routes.
One-Step Synthesis Focus: Specifically designed for one-step synthesis, it provides concise and direct routes for your target compounds, streamlining the synthesis process.
Accurate Predictions: Utilizing the extensive PISTACHIO, BKMS_METABOLIC, PISTACHIO_RINGBREAKER, REAXYS, REAXYS_BIOCATALYSIS database, our tool offers high-accuracy predictions, reflecting the latest in chemical research and data.
Strategy Settings
Precursor scoring | Relevance Heuristic |
---|---|
Min. plausibility | 0.01 |
Model | Template_relevance |
Template Set | Pistachio/Bkms_metabolic/Pistachio_ringbreaker/Reaxys/Reaxys_biocatalysis |
Top-N result to add to graph | 6 |
Feasible Synthetic Routes
Disclaimer and Information on In-Vitro Research Products
Please be aware that all articles and product information presented on BenchChem are intended solely for informational purposes. The products available for purchase on BenchChem are specifically designed for in-vitro studies, which are conducted outside of living organisms. In-vitro studies, derived from the Latin term "in glass," involve experiments performed in controlled laboratory settings using cells or tissues. It is important to note that these products are not categorized as medicines or drugs, and they have not received approval from the FDA for the prevention, treatment, or cure of any medical condition, ailment, or disease. We must emphasize that any form of bodily introduction of these products into humans or animals is strictly prohibited by law. It is essential to adhere to these guidelines to ensure compliance with legal and ethical standards in research and experimentation.