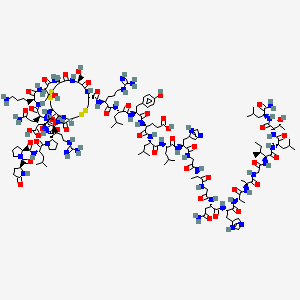
Orexin A
- Click on QUICK INQUIRY to receive a quote from our team of experts.
- With the quality product at a COMPETITIVE price, you can focus more on your research.
Overview
Description
Orexin A (OxA), also known as hypocretin-1, is a 33-amino-acid neuropeptide derived from the prepro-orexin precursor. It is synthesized exclusively in the lateral hypothalamus and plays critical roles in regulating arousal, sleep-wake cycles, appetite, and reward pathways . This compound binds to two G-protein-coupled receptors, OX1R and OX2R, with nearly equal affinity (OX1R: Kd ≈ 20–30 nM; OX2R: Kd ≈ 30–40 nM) . Its structural stability is attributed to two intramolecular disulfide bonds, which distinguish it from the shorter, linear Orexin B (OxB) . Dysregulation of orexin signaling is implicated in narcolepsy, insomnia, addiction, and mood disorders .
Preparation Methods
Synthetic Routes and Reaction Conditions: Orexin A is synthesized through a series of peptide bond formations. The process involves solid-phase peptide synthesis (SPPS), where amino acids are sequentially added to a growing peptide chain anchored to a solid resin. The reaction conditions typically involve the use of coupling reagents like N,N’-diisopropylcarbodiimide (DIC) and hydroxybenzotriazole (HOBt) to facilitate peptide bond formation. The final product is cleaved from the resin and purified using high-performance liquid chromatography (HPLC) .
Industrial Production Methods: Industrial production of this compound involves recombinant DNA technology. The gene encoding this compound is inserted into an expression vector, which is then introduced into a host organism, such as Escherichia coli or yeast. The host organism produces this compound, which is subsequently purified using chromatographic techniques .
Chemical Reactions Analysis
Structural Stability and Disulfide Bond Formation
Orexin A contains four cysteine residues forming two disulfide bonds (Cys6–Cys12 and Cys7–Cys14), which stabilize its tertiary structure . These bonds confer resistance to proteolytic degradation and enable selective receptor binding. Reduction of these bonds disrupts receptor activation, as confirmed by mutagenesis studies .
Key Data :
-
Structural impact : Circular dichroism shows α-helical segments (residues 6–14 and 18–33) stabilized by disulfide bonds .
Receptor Binding and Selectivity
This compound binds both OX1R and OX2R receptors, but with differential selectivity governed by specific residues:
-
OX1R binding : Thr111²·⁶⁰ and Gln134³·³² form hydrogen bonds with the peptide’s C-terminal residues (T32 and L33) .
-
OX2R binding : A127³·³³ (mutated to Thr in OX1R) creates a steric clash, reducing OX1R affinity .
Ligand-Receptor Interaction Table :
Receptor | Key Binding Residues | Interaction Type | Affinity (pKi) |
---|---|---|---|
OX1R | Thr111²·⁶⁰, Gln134³·³² | Hydrogen bonding | 8.7–9.4 |
OX2R | His350⁷·³⁸, Tyr317⁶·⁴⁸ | Hydrophobic/π-stacking | 7.2–9.7 |
Intracellular Signaling Pathways
This compound activates Gq and Gi proteins, triggering cascades via phospholipases and adenylyl cyclases :
-
Gq coupling : Stimulates phospholipase C (PLC), increasing IP3 and cytosolic Ca²⁺ .
-
Gi coupling : Inhibits adenylyl cyclase, reducing cAMP in cortical neurons .
Enzymatic Modulation :
Enzyme | Pathway | Effect of this compound |
---|---|---|
Phospholipase C | IP3/DAG cascade | ↑ Activity |
Adenylyl cyclase | cAMP synthesis | ↓ (OX2R) or ↑ (OX1R) |
Calcium Dynamics
This compound induces biphasic Ca²⁺ signaling:
Calcium Response Parameters :
Analytical Detection and Quantification
A mass spectrometry-based MRM assay achieves high sensitivity for this compound :
Workflow :
-
Sample prep : Immunoaffinity enrichment.
-
Digestion : Trypsin/Lys-C cleavage.
-
Detection : Quantify via transitions m/z 641.8→706.4 (orexin-A) and m/z 646.3→711.4 (stable isotope standard) .
Conformational Dynamics
Molecular dynamics simulations reveal two binding modes for this compound in OX2R :
-
Extended α-helix : Stabilized by hydrogen bonds with Gln134³·³² and Tyr317⁶·⁴⁸.
-
Bent conformation : Binds TM5/TM7 residues, enhancing receptor activation .
Key Interactions :
Scientific Research Applications
Introduction to Orexin A
This compound, a neuropeptide produced in the lateral hypothalamus, plays a crucial role in regulating various physiological functions, particularly those related to arousal, sleep-wake cycles, and energy homeostasis. The discovery of this compound has led to significant research into its applications in treating sleep disorders, obesity, and neurodegenerative diseases. This article aims to provide a comprehensive overview of the applications of this compound, supported by data tables and documented case studies.
Physiological Functions of this compound
This compound operates primarily through two receptor subtypes: OX1 and OX2. Its physiological roles include:
- Regulation of Sleep-Wake Cycles : this compound promotes wakefulness and inhibits rapid eye movement (REM) sleep. Studies show that administration of this compound leads to increased wakefulness and reduced sleep duration in animal models .
- Energy Expenditure : this compound is linked to increased energy expenditure and spontaneous physical activity. It has been shown to enhance metabolic rates without affecting food intake .
- Modulation of Neurotransmitter Release : this compound influences the release of neurotransmitters such as histamine, which is implicated in arousal mechanisms .
Insomnia Treatment
Orexin receptor antagonists have emerged as a promising treatment for insomnia. By blocking the action of this compound, these drugs can promote sleep without the side effects associated with traditional hypnotics. Clinical trials have demonstrated that orexin antagonists effectively improve sleep quality in patients with insomnia .
Narcolepsy Management
Narcolepsy, characterized by excessive daytime sleepiness and cataplexy, is closely linked to the loss of orexin-producing neurons. Replacement therapies targeting orexin signaling are being explored as potential treatments for narcolepsy. Studies involving this compound administration in animal models have shown improvements in wakefulness and reductions in sleep episodes .
Role in Neurodegenerative Diseases
Recent research indicates that this compound may play a protective role in neurodegenerative diseases such as Alzheimer's disease and Lewy body dementia. Its neuroprotective effects are attributed to its ability to enhance neuronal survival under stress conditions . Case studies have reported altered levels of this compound in patients with Lewy body disease, suggesting its potential as a biomarker for disease progression .
Table 1: Summary of Key Findings on this compound Applications
Table 2: Effects of this compound on Sleep-Wake Cycle
Dose (μg/rat) | Effect on Wakefulness (%) | Effect on REM Sleep (%) | Latency to REM Sleep (minutes) |
---|---|---|---|
1 | +20 | -10 | +5 |
10 | +40 | -30 | +10 |
30 | +60 | -50 | +15 |
Mechanism of Action
Orexin A exerts its effects by binding to and activating two G-protein-coupled receptors, orexin receptor type 1 (OX1R) and orexin receptor type 2 (OX2R). Upon binding, this compound triggers a cascade of intracellular signaling pathways, including the activation of adenylate cyclase, which increases cyclic adenosine monophosphate (cAMP) levels. This leads to the activation of protein kinase A (PKA) and subsequent phosphorylation of target proteins. This compound also stimulates the release of neurotransmitters such as dopamine, serotonin, and norepinephrine, promoting wakefulness and arousal .
Comparison with Similar Compounds
Orexin B (OxB)
Structural and Functional Differences :
- Sequence: OxB is a 28-amino-acid peptide lacking disulfide bonds, making it more susceptible to proteolytic degradation compared to OxA .
- Receptor Affinity : OxB binds OX2R with high affinity (Kd ≈ 30–50 nM) but has 100–1,000-fold lower affinity for OX1R (Kd ≈ 2–3 μM) .
- Physiological Effects :
- OxA induces stronger neuronal excitation in the prefrontal cortex at high doses (100 nM), while OxB shows comparable effects only at lower doses (10–30 nM) .
- OxA is more potent in regulating stress responses and addiction pathways, whereas OxB is critical for stabilizing sleep architecture via OX2R .
Table 1: Orexin A vs. Orexin B
Property | This compound (OxA) | Orexin B (OxB) |
---|---|---|
Amino Acid Length | 33 | 28 |
Disulfide Bonds | 2 | 0 |
OX1R Affinity (Kd) | 20–30 nM | 2–3 μM |
OX2R Affinity (Kd) | 30–40 nM | 30–50 nM |
Key Physiological Role | Stress, addiction, arousal | Sleep stability, energy balance |
Dual Orexin Receptor Antagonists (DORAs) :
- Suvorexant : The first FDA-approved DORA for insomnia. It inhibits OxA-induced locomotor activity (IC50: 0.55 nM for OX1R; 0.35 nM for OX2R) and reduces sleep latency .
- Compound 38b : A 1,3,5-trioxazatriquinan derivative with dual antagonism (OX1R IC50: 6.2 nM; OX2R IC50: 3.8 nM) and brain permeability .
Selective OX1R Antagonists (SORA1s) :
- Compound 41b : Exhibits >100-fold selectivity for OX1R (IC50: 1.4 nM) over OX2R (IC50: 150 nM). It suppresses OxA-mediated anxiety but has minimal impact on sleep .
Selective OX2R Antagonists (SORA2s) :
- L4 : A virtual screening-derived compound with OX2R antagonism (IC50: 2.2 μM). It shows promise for insomnia but lacks intrinsic activity .
Functional and Clinical Implications
- Therapeutic Selectivity : DORAs like suvorexant improve sleep maintenance but may cause next-day sedation. SORA1s (e.g., 41b) are better suited for anxiety and substance use disorders due to minimal sleep disruption .
- Receptor Distribution : OX1R is enriched in the prefrontal cortex and locus coeruleus (stress pathways), while OX2R dominates in the tuberomammillary nucleus (sleep regulation) . This explains OxA’s broader role in arousal and OxB’s sleep-specific effects.
- Pathological Context : In methamphetamine withdrawal, reduced OxA levels correlate with depressive symptoms, highlighting its role in mood regulation .
Biological Activity
Orexin A, also known as hypocretin-1, is a neuropeptide produced in the hypothalamus that plays a crucial role in regulating various physiological functions. It is best known for its involvement in the regulation of sleep-wake cycles, appetite control, energy metabolism, and neuroendocrine functions. The discovery of this compound in the late 1990s has led to extensive research into its biological activity and its implications for health and disease.
1. Regulation of Feeding Behavior
This compound is integral to the control of feeding behavior. Studies have demonstrated that this compound stimulates appetite and promotes food intake. For instance, Monda et al. (2003) found that administering this compound to fasting rats resulted in increased food consumption when food was presented after a delay, highlighting its role in meal initiation and energy balance .
Table 1: Effects of this compound on Feeding Behavior
Study | Methodology | Findings |
---|---|---|
Monda et al. (2003) | Intracerebroventricular injection | Increased food intake post-injection |
Tsuneki et al. (2010) | Glucose metabolism assays | Enhanced glucose uptake and production |
DiRocco & Grill (1979) | Decerebrated rat models | Maintained glucose control despite brain injury |
2. Impact on Energy Metabolism
This compound influences energy homeostasis by modulating metabolic pathways. It has been shown to enhance glucose production in the liver and facilitate glucose uptake in skeletal muscle . The orexinergic system responds to fluctuations in glucose levels, which suggests that it plays a role in maintaining energy balance according to dietary intake.
3. Arousal and Wakefulness
This compound is critically involved in promoting wakefulness and arousal. It activates neurons in the locus coeruleus, increasing noradrenergic cell firing, which enhances alertness and physical activity . This function is particularly relevant in understanding sleep disorders such as narcolepsy, where orexin-producing neurons are destroyed.
Table 2: this compound's Role in Arousal
Function | Mechanism | Outcome |
---|---|---|
Increases arousal | Activates locus coeruleus | Enhanced alertness and locomotor activity |
Modulates neuroendocrine function | Influences hormone release | Regulates stress response and metabolism |
4. Thermoregulation
This compound also plays a role in thermoregulation. It has been observed that this compound administration can elevate body temperature, which may be linked to its effects on energy expenditure and metabolic rate . This thermogenic effect suggests a potential mechanism by which this compound contributes to maintaining homeostasis during periods of fasting or cold exposure.
Case Study 1: Narcolepsy
Research has identified a significant link between orexin deficiency and narcolepsy with cataplexy. Individuals with this condition often exhibit low levels of this compound due to the loss of hypothalamic neurons that produce it. This deficiency leads to disrupted sleep-wake cycles and excessive daytime sleepiness .
Case Study 2: Obesity Models
In transgenic mouse models lacking orexin-producing neurons, researchers observed obesity despite lower caloric intake compared to wild-type mice. This finding underscores the importance of this compound in regulating not just appetite but also energy expenditure through spontaneous physical activity (SPA) .
Q & A
Basic Research Questions
Q. What experimental techniques are used to quantify Orexin A levels in brain tissue?
- Methodological Answer : this compound quantification typically involves immunoassays (e.g., ELISA) or high-performance liquid chromatography coupled with mass spectrometry (HPLC-MS). For tissue-specific localization, immunohistochemistry (IHC) using validated antibodies is standard. Critical steps include validating antibody specificity via knockout controls and normalizing results to protein concentration or housekeeping genes .
Q. How is this compound’s role in arousal regulation studied in rodent models?
- Methodological Answer : Rodent studies employ EEG/EMG recordings to correlate this compound levels with sleep-wake cycles. Microinjection of this compound into hypothalamic regions (e.g., locus coeruleus) combined with behavioral assays (e.g., open-field tests) can assess arousal. Control groups should include Orexin receptor antagonists (e.g., SB-334867) to isolate effects .
Q. What are standard protocols for validating this compound antibodies in immunohistochemistry?
- Methodological Answer : Validation requires (1) pre-adsorption controls (antibody incubated with excess this compound peptide), (2) comparison with knockout tissue, and (3) cross-reactivity tests against structurally similar peptides (e.g., Orexin B). Reproducibility should be confirmed across multiple tissue sections and labs .
Advanced Research Questions
Q. How can researchers address discrepancies in this compound’s effects on feeding behavior across animal models?
- Methodological Answer : Variability may arise from species-specific receptor expression (OX1R vs. OX2R) or experimental conditions (fasting duration, diet composition). Mitigation strategies include standardized metabolic cages for consistent feeding measurements, transcriptomic profiling of receptor subtypes, and meta-analyses of published datasets to identify confounding variables .
Q. What strategies optimize this compound detection in plasma given its low concentration and instability?
- Methodological Answer : Pre-analytical steps are critical: use EDTA-coated tubes to inhibit proteolysis, immediate centrifugation, and storage at -80°C. Enrichment techniques like immunoprecipitation or solid-phase extraction improve sensitivity. Parallel measurement of stable metabolites (e.g., this compound (12-28)) can validate degradation rates .
Q. How do in vivo optogenetic approaches elucidate this compound’s site-specific neuromodulatory effects?
- Methodological Answer : Optogenetic activation/inhibition of Orexin-producing neurons (e.g., in lateral hypothalamus) combined with fiber photometry allows real-time monitoring of this compound release. Pairing with behavioral paradigms (e.g., fear conditioning) and calcium imaging in downstream targets (e.g., amygdala) reveals circuit-specific roles .
Q. What statistical methods reconcile contradictory findings on this compound’s receptor affinity?
- Methodological Answer : Competitive binding assays (e.g., radioligand displacement) with curve-fitting models (e.g., Hill equation) quantify receptor affinity. Bayesian meta-analysis can pool data from disparate studies, weighting for sample size and methodological rigor. In silico docking simulations further predict binding dynamics under varying pH or co-factor conditions .
Q. Data Contradiction and Reproducibility
Q. Why do studies report conflicting results on this compound’s role in stress responses?
- Methodological Answer : Differences in stress induction protocols (acute vs. chronic), sampling timepoints, or circadian phases may drive inconsistencies. Standardizing stress paradigms (e.g., forced swim test duration) and controlling for diurnal this compound fluctuations (via timed euthanasia) enhance reproducibility .
Q. How can researchers improve cross-lab reproducibility in this compound knockout studies?
- Methodological Answer : Use publicly available knockout strains (e.g., B6;129S-Hcrt<tm1Lex>) with genotyping protocols. Report detailed housing conditions (e.g., light cycles, diet) and share raw data via repositories like Figshare. Collaborative inter-lab studies using identical protocols reduce technical variability .
Q. Innovative Methodologies
Q. What emerging technologies advance this compound research in heterogeneous brain tissues?
- Methodological Answer : Single-cell RNA sequencing identifies this compound-expressing neuron subpopulations. Spatial transcriptomics (e.g., 10x Genomics Visium) maps peptide distribution across hypothalamic nuclei. CRISPR-Cas9 editing in organoids models receptor signaling pathways in human cells .
Q. Tables for Key Methodological Comparisons
Technique | Application | Advantages | Limitations |
---|---|---|---|
ELISA | Quantifying this compound in plasma | High throughput, cost-effective | Cross-reactivity with degraded peptides |
HPLC-MS | Absolute quantification in brain tissue | High specificity, detects metabolites | Requires specialized equipment |
Optogenetics + Fiber Photometry | Real-time this compound dynamics in vivo | Spatiotemporal precision | Invasive, technically challenging |
Q. Citations and Quality Assurance
Properties
CAS No. |
205599-75-3 |
---|---|
Molecular Formula |
C152H243N47O44S4 |
Molecular Weight |
3561.1 g/mol |
IUPAC Name |
(4S)-5-[[(2S)-1-[[(2S)-1-[[(2S)-1-[[2-[[(2S)-1-[[2-[[(2S)-4-amino-1-[[(2S)-1-[[(2S)-1-[[(2S)-1-[[2-[[(2S,3S)-1-[[(2S)-1-[[(2S,3R)-1-[[(2S)-1-amino-4-methyl-1-oxopentan-2-yl]amino]-3-hydroxy-1-oxobutan-2-yl]amino]-4-methyl-1-oxopentan-2-yl]amino]-3-methyl-1-oxopentan-2-yl]amino]-2-oxoethyl]amino]-1-oxopropan-2-yl]amino]-1-oxopropan-2-yl]amino]-3-(1H-imidazol-5-yl)-1-oxopropan-2-yl]amino]-1,4-dioxobutan-2-yl]amino]-2-oxoethyl]amino]-1-oxopropan-2-yl]amino]-2-oxoethyl]amino]-3-(1H-imidazol-5-yl)-1-oxopropan-2-yl]amino]-4-methyl-1-oxopentan-2-yl]amino]-4-methyl-1-oxopentan-2-yl]amino]-4-[[(2S)-2-[[(2S)-2-[[(2S)-2-[[(1R,4S,7S,10S,13S,16R,21R,24S,31R)-7-(4-aminobutyl)-10-(3-amino-3-oxopropyl)-13-(3-carbamimidamidopropyl)-31-[[(2S)-3-carboxy-2-[[(2S)-1-[(2S)-4-methyl-2-[[(2S)-1-[(2S)-5-oxopyrrolidine-2-carbonyl]pyrrolidine-2-carbonyl]amino]pentanoyl]pyrrolidine-2-carbonyl]amino]propanoyl]amino]-4-[(1R)-1-hydroxyethyl]-24-(hydroxymethyl)-3,6,9,12,15,23,26,32-octaoxo-18,19,28,29-tetrathia-2,5,8,11,14,22,25,33-octazabicyclo[14.10.7]tritriacontane-21-carbonyl]amino]-5-carbamimidamidopentanoyl]amino]-4-methylpentanoyl]amino]-3-(4-hydroxyphenyl)propanoyl]amino]-5-oxopentanoic acid |
InChI |
InChI=1S/C152H243N47O44S4/c1-20-76(14)118(146(239)188-96(51-74(10)11)138(231)197-119(80(18)201)147(240)180-92(121(156)214)47-70(2)3)195-115(209)62-166-123(216)78(16)171-124(217)79(17)172-131(224)99(55-84-59-162-69-169-84)186-136(229)100(56-111(155)205)174-114(208)61-165-122(215)77(15)170-113(207)60-167-125(218)98(54-83-58-161-68-168-83)185-134(227)95(50-73(8)9)183-132(225)93(48-71(4)5)182-129(222)90(38-41-116(210)211)179-135(228)97(53-82-32-34-85(203)35-33-82)184-133(226)94(49-72(6)7)181-127(220)88(29-24-44-164-152(159)160)177-140(233)104-64-244-245-65-105-141(234)176-87(28-23-43-163-151(157)158)126(219)178-89(36-39-110(154)204)128(221)175-86(27-21-22-42-153)130(223)196-120(81(19)202)148(241)194-107(142(235)190-103(63-200)139(232)192-104)67-247-246-66-106(143(236)193-105)191-137(230)101(57-117(212)213)187-144(237)108-30-26-46-199(108)150(243)102(52-75(12)13)189-145(238)109-31-25-45-198(109)149(242)91-37-40-112(206)173-91/h32-35,58-59,68-81,86-109,118-120,200-203H,20-31,36-57,60-67,153H2,1-19H3,(H2,154,204)(H2,155,205)(H2,156,214)(H,161,168)(H,162,169)(H,165,215)(H,166,216)(H,167,218)(H,170,207)(H,171,217)(H,172,224)(H,173,206)(H,174,208)(H,175,221)(H,176,234)(H,177,233)(H,178,219)(H,179,228)(H,180,240)(H,181,220)(H,182,222)(H,183,225)(H,184,226)(H,185,227)(H,186,229)(H,187,237)(H,188,239)(H,189,238)(H,190,235)(H,191,230)(H,192,232)(H,193,236)(H,194,241)(H,195,209)(H,196,223)(H,197,231)(H,210,211)(H,212,213)(H4,157,158,163)(H4,159,160,164)/t76-,77-,78-,79-,80+,81+,86-,87-,88-,89-,90-,91-,92-,93-,94-,95-,96-,97-,98-,99-,100-,101-,102-,103-,104-,105-,106-,107-,108-,109-,118-,119-,120-/m0/s1 |
InChI Key |
OFNHNCAUVYOTPM-IIIOAANCSA-N |
Isomeric SMILES |
CC[C@H](C)[C@@H](C(=O)N[C@@H](CC(C)C)C(=O)N[C@@H]([C@@H](C)O)C(=O)N[C@@H](CC(C)C)C(=O)N)NC(=O)CNC(=O)[C@H](C)NC(=O)[C@H](C)NC(=O)[C@H](CC1=CN=CN1)NC(=O)[C@H](CC(=O)N)NC(=O)CNC(=O)[C@H](C)NC(=O)CNC(=O)[C@H](CC2=CN=CN2)NC(=O)[C@H](CC(C)C)NC(=O)[C@H](CC(C)C)NC(=O)[C@H](CCC(=O)O)NC(=O)[C@H](CC3=CC=C(C=C3)O)NC(=O)[C@H](CC(C)C)NC(=O)[C@H](CCCNC(=N)N)NC(=O)[C@@H]4CSSC[C@H]5C(=O)N[C@H](C(=O)N[C@H](C(=O)N[C@H](C(=O)N[C@H](C(=O)N[C@@H](CSSC[C@@H](C(=O)N5)NC(=O)[C@H](CC(=O)O)NC(=O)[C@@H]6CCCN6C(=O)[C@H](CC(C)C)NC(=O)[C@@H]7CCCN7C(=O)[C@@H]8CCC(=O)N8)C(=O)N[C@H](C(=O)N4)CO)[C@@H](C)O)CCCCN)CCC(=O)N)CCCNC(=N)N |
Canonical SMILES |
CCC(C)C(C(=O)NC(CC(C)C)C(=O)NC(C(C)O)C(=O)NC(CC(C)C)C(=O)N)NC(=O)CNC(=O)C(C)NC(=O)C(C)NC(=O)C(CC1=CN=CN1)NC(=O)C(CC(=O)N)NC(=O)CNC(=O)C(C)NC(=O)CNC(=O)C(CC2=CN=CN2)NC(=O)C(CC(C)C)NC(=O)C(CC(C)C)NC(=O)C(CCC(=O)O)NC(=O)C(CC3=CC=C(C=C3)O)NC(=O)C(CC(C)C)NC(=O)C(CCCNC(=N)N)NC(=O)C4CSSCC5C(=O)NC(C(=O)NC(C(=O)NC(C(=O)NC(C(=O)NC(CSSCC(C(=O)N5)NC(=O)C(CC(=O)O)NC(=O)C6CCCN6C(=O)C(CC(C)C)NC(=O)C7CCCN7C(=O)C8CCC(=O)N8)C(=O)NC(C(=O)N4)CO)C(C)O)CCCCN)CCC(=O)N)CCCNC(=N)N |
Related CAS |
205640-90-0 (human) |
Origin of Product |
United States |
Disclaimer and Information on In-Vitro Research Products
Please be aware that all articles and product information presented on BenchChem are intended solely for informational purposes. The products available for purchase on BenchChem are specifically designed for in-vitro studies, which are conducted outside of living organisms. In-vitro studies, derived from the Latin term "in glass," involve experiments performed in controlled laboratory settings using cells or tissues. It is important to note that these products are not categorized as medicines or drugs, and they have not received approval from the FDA for the prevention, treatment, or cure of any medical condition, ailment, or disease. We must emphasize that any form of bodily introduction of these products into humans or animals is strictly prohibited by law. It is essential to adhere to these guidelines to ensure compliance with legal and ethical standards in research and experimentation.