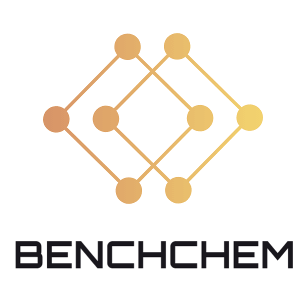
T-2 Toxin-13C24
- Click on QUICK INQUIRY to receive a quote from our team of experts.
- With the quality product at a COMPETITIVE price, you can focus more on your research.
Overview
Description
T-2 Toxin-13C24 is a fully 13C-substituted analog of T-2 toxin, a trichothecene mycotoxin produced by various Fusarium species. This compound is primarily used as an internal standard for the quantification of T-2 toxin in cereals using high-performance liquid chromatography coupled with tandem mass spectrometry (HPLC-MS/MS) . T-2 toxin is known for its potent inhibitory effects on eukaryotic protein synthesis and has significant toxicological implications .
Preparation Methods
Synthetic Routes and Reaction Conditions: The synthesis of T-2 Toxin-13C24 involves the incorporation of 13C isotopes into the T-2 toxin molecule. This process typically requires high-resolution mass spectrometry, 1H-NMR, 13C-NMR, and tandem mass spectrometry to confirm the structure and identity of the compound . The isotopic distribution of this compound indicates a total isotopic enrichment of approximately 98.2% .
Industrial Production Methods: Industrial production of this compound involves the use of stable isotope dilution mass spectrometry (IDMS) techniques. This method allows for the precise quantification of T-2 toxin in various matrices, such as maize and oats, by compensating for matrix enhancement effects through the application of the isotope-substituted internal standard .
Chemical Reactions Analysis
Types of Reactions: T-2 Toxin-13C24 undergoes various chemical reactions, including oxidation, reduction, and substitution. These reactions are essential for its detection and quantification in different analytical methods.
Common Reagents and Conditions: Common reagents used in the analysis of this compound include acetonitrile, methanol, and water. The compound is often subjected to conditions such as evaporation under a gentle stream of nitrogen and direct injection into ion sources for mass spectrometry .
Major Products Formed: The major products formed from the reactions of this compound include its metabolites, such as HT-2 toxin. These metabolites are crucial for understanding the toxicological effects and metabolic pathways of the compound .
Scientific Research Applications
T-2 Toxin-13C24 has a wide range of scientific research applications, particularly in the fields of chemistry, biology, medicine, and industry. It is used as an internal standard for the quantification of T-2 toxin in cereals, which is essential for food safety and regulatory compliance . Additionally, it plays a significant role in studying the toxicological effects of T-2 toxin and its metabolites on various biological systems .
Mechanism of Action
The mechanism of action of T-2 Toxin-13C24 is primarily based on its ability to inhibit protein synthesis in eukaryotic cells. This inhibition occurs through the binding of the toxin to the peptidyltransferase enzyme in the 60S ribosomal subunit, leading to a ribotoxic stress response that triggers signaling pathways such as JNK and p38 MAPK . The presence of the epoxide group at the C12,13 position is crucial for its toxicological activity .
Comparison with Similar Compounds
Similar Compounds: Similar compounds to T-2 Toxin-13C24 include other trichothecene mycotoxins such as HT-2 toxin, 3’-hydroxy-T-2 toxin, and 4’-hydroxy-T-2 toxin . These compounds share similar toxicological properties and metabolic pathways.
Uniqueness: this compound is unique due to its complete 13C substitution, which makes it an ideal internal standard for analytical methods. This isotopic labeling allows for precise quantification and compensation for matrix effects in various analytical techniques .
properties
Molecular Formula |
C24H34O9 |
---|---|
Molecular Weight |
490.35 g/mol |
IUPAC Name |
[(1'S,2S,2'R,4'S,7'R,9'R,10'R,11'S)-11'-acetyloxy-2'-(acetyloxy(113C)methyl)-10'-hydroxy-1',5'-di((113C)methyl)spiro[(2,3-13C2)oxirane-2,12'-8-oxatricyclo[7.2.1.02,7]dodec-5-ene]-4'-yl] 3-(113C)methyl(1,2,3,4-13C4)butanoate |
InChI |
InChI=1S/C24H34O9/c1-12(2)7-18(27)32-16-9-23(10-29-14(4)25)17(8-13(16)3)33-21-19(28)20(31-15(5)26)22(23,6)24(21)11-30-24/h8,12,16-17,19-21,28H,7,9-11H2,1-6H3/t16-,17+,19+,20+,21+,22+,23+,24-/m0/s1/i1+1,2+1,3+1,4+1,5+1,6+1,7+1,8+1,9+1,10+1,11+1,12+1,13+1,14+1,15+1,16+1,17+1,18+1,19+1,20+1,21+1,22+1,23+1,24+1 |
InChI Key |
BXFOFFBJRFZBQZ-SOROUPBBSA-N |
Isomeric SMILES |
[13CH3][13C]1=[13CH][13C@@H]2[13C@]([13CH2][13C@@H]1O[13C](=O)[13CH2][13CH]([13CH3])[13CH3])([13C@]3([13C@@H]([13C@H]([13C@H]([13C@@]34[13CH2]O4)O2)O)O[13C](=O)[13CH3])[13CH3])[13CH2]O[13C](=O)[13CH3] |
Canonical SMILES |
CC1=CC2C(CC1OC(=O)CC(C)C)(C3(C(C(C(C34CO4)O2)O)OC(=O)C)C)COC(=O)C |
Origin of Product |
United States |
Disclaimer and Information on In-Vitro Research Products
Please be aware that all articles and product information presented on BenchChem are intended solely for informational purposes. The products available for purchase on BenchChem are specifically designed for in-vitro studies, which are conducted outside of living organisms. In-vitro studies, derived from the Latin term "in glass," involve experiments performed in controlled laboratory settings using cells or tissues. It is important to note that these products are not categorized as medicines or drugs, and they have not received approval from the FDA for the prevention, treatment, or cure of any medical condition, ailment, or disease. We must emphasize that any form of bodily introduction of these products into humans or animals is strictly prohibited by law. It is essential to adhere to these guidelines to ensure compliance with legal and ethical standards in research and experimentation.