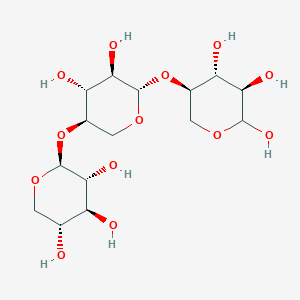
Xylotriose
- Click on QUICK INQUIRY to receive a quote from our team of experts.
- With the quality product at a COMPETITIVE price, you can focus more on your research.
Overview
Description
Xylotriose (XOS3), a xylooligosaccharide (XOS) composed of three β-1,4-linked xylose units, is a hydrolysis product of xylan, a major hemicellulose component in plant biomass . It is widely recognized for its prebiotic properties, promoting gut health by modulating microbiota and enhancing short-chain fatty acid (SCFA) production . Industrially, this compound is valorized in food, pharmaceutical, and cosmetic sectors due to its bioactivity, low calorific value, and stability under processing conditions . Its production primarily involves enzymatic hydrolysis of xylan using endo-1,4-β-xylanases, though yield optimization remains a challenge due to competing degradation pathways .
Preparation Methods
Synthetic Routes and Reaction Conditions: Xylotriose can be synthesized through the enzymatic hydrolysis of xylan, a major component of plant cell walls. The process involves the use of xylanase enzymes, which cleave the β-1,4-glycosidic bonds in xylan to produce xylooligosaccharides, including this compound. The reaction conditions typically include a pH range of 4.5 to 6.5 and temperatures between 40°C and 60°C .
Industrial Production Methods: Industrial production of this compound often involves the use of agricultural residues such as corncobs, sugarcane bagasse, and rice straw. These materials are pretreated to extract xylan, which is then subjected to enzymatic hydrolysis. The resulting mixture is purified using techniques such as silica gel column chromatography to isolate this compound .
Chemical Reactions Analysis
Acid-Catalyzed Hydrolysis
Xylotriose undergoes acid hydrolysis under conditions optimized for xylooligosaccharide (XOS) production. Key findings include:
-
Optimal conditions : 1% sulfuric acid at 120°C for 60 minutes yielded 45.18% total XOS, with this compound contributing 13% of the product mixture .
-
Degradation pathways : Prolonged reaction times (>60 min) or higher temperatures (>120°C) led to further breakdown into xylose, reducing XOS purity .
Condition | This compound Yield (%) | Xylose Yield (%) |
---|---|---|
1% H₂SO₄, 120°C, 60 min | 13 | 18.78 |
1% H₂SO₄, 135°C, 60 min | 7 | 66.13 |
Enzymatic Hydrolysis
Xylanases selectively cleave this compound into shorter oligosaccharides or xylose:
-
Endo-xylanase activity : Enzymes from Aspergillus terreus and Thermoanaerobacterium sp. predominantly yield xylobiose (X2) and this compound (X3) at optimal pH (4.5–6.1) and temperature (35–65°C) .
-
Substrate inhibition : High this compound concentrations (>4 mM) inhibit β-xylosidases, reducing hydrolysis efficiency .
Kinetic parameters for β-xylosidase (GH52 family) :
Substrate | Kₘ (mM) | kₐₐₜ (s⁻¹) | Binding ΔH (kJ/mol) |
---|---|---|---|
Xylobiose | 0.32 | 2.1 | -38.5 |
This compound | 1.15 | 1.8 | -29.7 |
Data from isothermal titration calorimetry (ITC) revealed tighter binding of xylobiose compared to this compound .
Pyrolysis Mechanisms
Fast pyrolysis of this compound at 500–700°C produces fragmentation products via:
-
Retro-aldol condensation : Loss of ethenediol forms β-D-xylopyranosylglyceraldehyde, which further decomposes to β-D-xylopyranosylglycolaldehyde .
-
Glycosidic bond cleavage : Maccoll elimination and pinacol ring contraction pathways generate anhydrosugars and volatile organic compounds .
Key pyrolysis products :
-
β-D-xylopyranosylglyceraldehyde
-
Formaldehyde
-
Glycolaldehyde derivatives
Stability Under Acidic and Alkaline Conditions
This compound stability varies significantly with pH and temperature:
-
Acidic conditions (pH 2–3) : Minimal decomposition (1–4% over 60 min at 100°C) .
-
Alkaline conditions (pH 11) : Rapid degradation (73% decomposition in 60 min at 100°C) via "alkaline peeling" reactions .
Condition | Decomposition (%) | Mechanism |
---|---|---|
pH 2.0, 100°C, 60 min | 21 | Acid-catalyzed hydrolysis |
pH 11.0, 100°C, 60 min | 73 | Alkaline peeling |
Industrial Production and Purification
This compound is co-produced with other XOS via hydrothermal pretreatment of lignocellulosic biomass:
-
Two-step process :
This compound’s reactivity is governed by its glycosidic linkages and hydroxyl group arrangement, enabling applications in food, pharmaceuticals, and biofuels. Further optimization of enzymatic and thermal processes could enhance its industrial scalability .
Scientific Research Applications
Nutritional Applications
Prebiotic Potential
Xylotriose exhibits prebiotic properties, promoting the growth of beneficial gut bacteria such as bifidobacteria and lactobacilli. This beneficial effect enhances gut health and improves nutrient absorption, particularly calcium . Studies have shown that this compound is not hydrolyzed in the upper gastrointestinal tract, allowing it to selectively stimulate colonic bacteria .
Table 1: Prebiotic Effects of this compound
Study Reference | Effect Observed | Organism Targeted |
---|---|---|
Increased bifidobacteria populations | Bifidobacterium spp. | |
Enhanced calcium absorption | Human subjects | |
Stimulated lactobacilli growth | Lactobacillus spp. |
Biomedical Applications
Antioxidant Activity
Research indicates that this compound possesses significant antioxidant properties, which can combat oxidative stress in human cells. This activity is dose-dependent and attributed to its ability to scavenge free radicals effectively . The antioxidant potential of this compound positions it as a candidate for therapeutic applications in managing diseases associated with oxidative stress.
Case Study: Antioxidant Efficacy
A study demonstrated that this compound exhibited up to 74% antioxidant activity at a concentration of 6 mg/ml when tested using the DPPH assay . This finding highlights its potential use in dietary supplements aimed at enhancing health through oxidative stress reduction.
Industrial Applications
Bioconversion Processes
this compound can be produced from lignocellulosic biomass through enzymatic hydrolysis using xylanases. This process is part of biorefinery strategies aimed at converting agricultural waste into value-added products such as bioethanol and organic acids . The enzymatic breakdown of hemicellulose into xylo-oligosaccharides like this compound is crucial for developing sustainable biofuels.
Table 2: Industrial Production Pathways for this compound
Process Type | Description | Reference |
---|---|---|
Enzymatic Hydrolysis | Use of xylanases to convert biomass into xylo-oligosaccharides | |
Fermentation | Conversion of xylose into bioethanol using yeast strains |
Agricultural Applications
Animal Feed Additive
this compound has been identified as a beneficial additive in animal feed, enhancing growth rates and disease resistance in livestock . Its prebiotic effects can improve gut health in animals, leading to better feed conversion ratios and overall productivity.
Cosmetic and Dermatological Uses
Skin Health Benefits
The antimicrobial and anti-inflammatory properties of this compound make it suitable for cosmetic applications. It has been reported to improve skin conditions by enhancing moisture retention and exhibiting protective effects against harmful microorganisms .
Mechanism of Action
Xylotriose exerts its effects primarily through its prebiotic properties. It is not digested by human enzymes and reaches the colon intact, where it is fermented by gut microbiota. This fermentation process produces short-chain fatty acids such as acetate, propionate, and butyrate, which have various health benefits, including improved gut barrier function and anti-inflammatory effects . The molecular targets include gut bacteria such as bifidobacteria and lactobacilli, which utilize this compound as a carbon source.
Comparison with Similar Compounds
Structural and Functional Comparison with Similar Compounds
Structural Differences
Xylotriose (DP3) differs from related XOS compounds in degree of polymerization (DP) and glycosidic linkages:
- Xylobiose (XOS2) : DP2, simplest XOS with two xylose units .
- Xylotetraose (XOS4) : DP4, less abundant due to rapid enzymatic hydrolysis to smaller units .
- Higher-DP XOS (DP5–6+) : Longer chains with reduced solubility and bioactivity compared to XOS2 and XOS3 .
Table 1: Structural Comparison of this compound with Similar XOS
Functional Properties
- Prebiotic Efficacy : XOS3 and XOS2 exhibit superior microbial selectivity compared to higher-DP XOS, stimulating Bifidobacterium and Lactobacillus growth .
- Bioavailability : XOS3 is less readily hydrolyzed by gut enzymes than XOS2, enabling sustained fermentation in the distal colon .
- Immunomodulation: In broiler studies, in ovo XOS3 administration increased plasma immunoglobulin levels more significantly than XOS2, suggesting DP-dependent immune activation .
Production and Degradation Dynamics
Enzymatic Hydrolysis Yields
This compound production is influenced by enzyme specificity, dosage, and reaction time:
- Endoxylanase Activity : GH10 and GH11 xylanases yield distinct XOS profiles. GH11 enzymes predominantly release XOS2 and XOS3, while GH10 enzymes produce longer XOS .
- Dosage Effects : Higher endoxylanase doses (2 U/mL) accelerate XOS3 hydrolysis into XOS2 and xylose, reducing XOS3 yields after 24 hours .
Table 2: this compound Production Under Different Enzymatic Conditions
Chemical Degradation
- FeCl3 Catalysis : At 180°C, 0.8% FeCl3 increases XOS3 degradation to 78% within 20 minutes, following first-order kinetics .
- pH Sensitivity : Acidic conditions accelerate XOS3 breakdown, though metal ions like Fe³⁺ enhance degradation beyond pH effects alone .
Challenges in Large-Scale Production
- Yield Optimization : Competing hydrolysis pathways limit XOS3 accumulation; enzyme cocktails with β-xylosidase inhibitors improve selectivity .
- Cost Efficiency: High-purity XOS3 requires costly purification steps, driving research into engineered enzymes and pretreatment technologies .
Functional Superiority Over Analogues
- Gut Health: XOS3 increases butyrate production 1.5-fold compared to XOS2 in vitro, correlating with enhanced colonocyte health .
- Thermostability : XOS3 retains functionality at high temperatures (180°C), making it suitable for heat-processed functional foods .
Biological Activity
Xylotriose, a xylo-oligosaccharide composed of three xylose units, has garnered attention for its diverse biological activities, particularly in the context of gut health, immunomodulation, and potential therapeutic applications. This article synthesizes current research findings, case studies, and data regarding the biological activity of this compound.
Overview of this compound
This compound is produced through the enzymatic hydrolysis of xylan, a major component of plant cell walls. It is classified as a prebiotic due to its ability to stimulate beneficial gut microbiota, particularly bifidobacteria. The structural formula for this compound is represented as:
where each "Xyl" denotes a xylose unit linked by β-(1→4) glycosidic bonds.
Prebiotic Effects
This compound exhibits significant prebiotic properties, enhancing the growth of beneficial gut bacteria. Research has shown that it selectively stimulates the proliferation of bifidobacteria in the human gut, leading to improved gut health and digestion. A study indicated that this compound could increase the population of Bifidobacterium species significantly compared to control groups without oligosaccharide supplementation .
Antimicrobial Activity
The antimicrobial properties of this compound have been documented against various pathogens. It has demonstrated high activity against bacteria such as Klebsiella pneumoniae, Enterococcus faecalis, and Pseudomonas aeruginosa. In vitro studies revealed that this compound exhibits an IC50 value of 0.45 mg/mL for DPPH scavenging activity, indicating its potential as an antioxidant agent .
Immunomodulatory Effects
This compound has been shown to modulate immune responses. It can reduce pro-inflammatory cytokine production in immune cells, contributing to its potential role in managing inflammatory diseases. A study highlighted that xylooligosaccharides (including this compound) could decrease cytokine levels in U-937 cells, suggesting a mechanism for its immunomodulatory effects .
Case Studies
- Gut Microbiota Modulation : A clinical trial involving participants supplemented with this compound showed a marked increase in bifidobacteria populations over four weeks, correlating with improved digestive health markers.
- Antioxidant Properties : In a laboratory setting, this compound was tested alongside other oligosaccharides for antioxidant activity. Results indicated that it outperformed many common antioxidants in scavenging free radicals.
- Cancer Research : Preliminary studies have suggested that xylooligosaccharides can inhibit tumor cell proliferation and induce apoptosis in cancer cell lines through modulation of glutathione homeostasis and interaction with Toll-like receptors (TLR4) .
Research Findings Summary
Q & A
Basic Research Questions
Q. What are the established methods for isolating Xylotriose from natural sources, and how can their efficiency be optimized?
- Methodological Answer : Isolation typically involves enzymatic or acid hydrolysis of xylan-rich biomass (e.g., agricultural residues). Optimization parameters include pH (4.0–6.0), temperature (40–60°C), and enzyme/substrate ratios. Post-hydrolysis, purification via gel filtration chromatography or HPLC is recommended. Efficiency can be assessed by yield (%) and purity (validated by NMR or mass spectrometry) .
Q. How is this compound structurally characterized, and what analytical techniques are most reliable for confirming its purity?
- Methodological Answer : Structural confirmation requires tandem techniques:
- NMR (¹H/¹³C for glycosidic linkage analysis),
- Mass Spectrometry (MALDI-TOF for molecular weight verification),
- HPLC (with refractive index/ELSD detectors for purity ≥95%). Cross-referencing with published spectral libraries ensures accuracy .
Q. What in vitro assays are suitable for evaluating this compound’s prebiotic activity, and how should controls be designed?
- Methodological Answer : Use anaerobic batch cultures with Bifidobacterium or Lactobacillus strains. Measure short-chain fatty acid (SCFA) production via GC-MS and bacterial growth (OD₆₀₀) over 24–48 hours. Controls must include blank media, non-prebiotic carbohydrates (e.g., glucose), and commercial prebiotics (e.g., FOS) for benchmarking .
Advanced Research Questions
Q. What experimental strategies can resolve contradictions in reported biological activities of this compound across studies?
- Methodological Answer : Conduct a systematic review with meta-analysis (PRISMA guidelines) to identify confounding variables (e.g., purity, assay conditions). Follow with controlled in vitro/in vivo replication studies, standardizing variables like dosage, microbial strains, and endpoint measurements. Apply the FINER framework (Feasible, Novel, Ethical, Relevant) to refine hypotheses .
Q. How can in silico modeling be integrated with experimental approaches to predict this compound interactions with microbial enzymes?
- Methodological Answer : Use molecular docking tools (AutoDock Vina, GROMACS) to model this compound binding to xylanases or ABC transporters. Validate predictions with enzymatic assays (e.g., Michaelis-Menten kinetics) and site-directed mutagenesis of key residues. Cross-validate with cryo-EM or X-ray crystallography data if available .
Q. What are the challenges in synthesizing this compound enzymatically versus chemically, and how can reaction conditions be standardized?
- Methodological Answer : Enzymatic synthesis (using xylosyltransferases) offers stereoselectivity but faces low yield (30–50%) due to side reactions. Chemical synthesis (e.g., Koenigs-Knorr glycosylation) requires toxic reagents (AgOTf) and complex purification. Standardization involves optimizing reaction time, solvent systems (e.g., DMF/H₂O), and immobilizing enzymes for reusability. Document protocols using MIABE standards for reproducibility .
Q. How do variations in xylan source (e.g., hardwood vs. grass) impact this compound yield and bioactivity?
- Methodological Answer : Perform comparative hydrolysis using xylan from birchwood (glucuronoxylan) vs. oat spelt (arabinoxylan). Quantify this compound yield via HPAEC-PAD and assess prebiotic activity (SCFA profiles) in identical microbial assays. Statistically analyze differences using ANOVA with post-hoc Tukey tests .
Q. Methodological Considerations
- Data Contradiction Analysis : Use funnel plots to detect publication bias in meta-analyses. For conflicting in vivo results, consider host microbiome variability and employ gnotobiotic models to isolate this compound effects .
- Reproducibility : Adhere to FAIR principles (Findable, Accessible, Interoperable, Reusable) by depositing raw data (e.g., NMR spectra, growth curves) in repositories like Zenodo or GlyTouCan .
Properties
CAS No. |
22416-59-7 |
---|---|
Molecular Formula |
C15H26O13 |
Molecular Weight |
414.36 g/mol |
IUPAC Name |
(2S,3R,4S,5R)-2-[(3R,4R,5R,6S)-4,5-dihydroxy-6-[(3R,4R,5R)-4,5,6-trihydroxyoxan-3-yl]oxyoxan-3-yl]oxyoxane-3,4,5-triol |
InChI |
InChI=1S/C15H26O13/c16-4-1-25-14(11(21)7(4)17)28-6-3-26-15(12(22)9(6)19)27-5-2-24-13(23)10(20)8(5)18/h4-23H,1-3H2/t4-,5-,6-,7+,8+,9+,10-,11-,12-,13?,14+,15+/m1/s1 |
InChI Key |
JCSJTDYCNQHPRJ-FDVJSPBESA-N |
Isomeric SMILES |
C1[C@H]([C@@H]([C@H]([C@@H](O1)O[C@@H]2CO[C@H]([C@@H]([C@H]2O)O)O[C@@H]3COC([C@@H]([C@H]3O)O)O)O)O)O |
Canonical SMILES |
C1C(C(C(C(O1)OC2COC(C(C2O)O)OC3COC(C(C3O)O)O)O)O)O |
Origin of Product |
United States |
Disclaimer and Information on In-Vitro Research Products
Please be aware that all articles and product information presented on BenchChem are intended solely for informational purposes. The products available for purchase on BenchChem are specifically designed for in-vitro studies, which are conducted outside of living organisms. In-vitro studies, derived from the Latin term "in glass," involve experiments performed in controlled laboratory settings using cells or tissues. It is important to note that these products are not categorized as medicines or drugs, and they have not received approval from the FDA for the prevention, treatment, or cure of any medical condition, ailment, or disease. We must emphasize that any form of bodily introduction of these products into humans or animals is strictly prohibited by law. It is essential to adhere to these guidelines to ensure compliance with legal and ethical standards in research and experimentation.