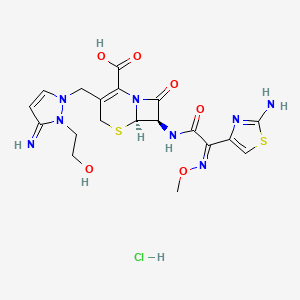
Cefoselis hydrochloride
- Click on QUICK INQUIRY to receive a quote from our team of experts.
- With the quality product at a COMPETITIVE price, you can focus more on your research.
Overview
Description
Cefoselis hydrochloride is a fourth-generation cephalosporin antibiotic. It is widely used in Japan and China for the clinical treatment of various gram-positive and gram-negative infections. This compound is particularly effective in treating respiratory and urinary tract infections .
Preparation Methods
Synthetic Routes and Reaction Conditions: The synthesis of cefoselis hydrochloride involves multiple steps. One common method starts with 7-aminocephalosporanic acid (7-ACA) as the core structure.
Industrial Production Methods: In industrial settings, the production of this compound often involves the use of macroporous resin in a fixed-bed column for separation and purification. The adsorption dynamics are predicted by the model of modified film-pore diffusion (MFPD), which helps in optimizing the key operating parameters such as initial adsorbate concentration, flow rate of import feed, and bed height of adsorbent .
Chemical Reactions Analysis
Types of Reactions: Cefoselis hydrochloride undergoes various chemical reactions, including:
Hydrolysis: The compound is prone to hydrolysis, especially in aqueous solutions.
Acid-Base Catalysis: General acid-base hydrolysis is observed in phosphate and acetate buffers.
Common Reagents and Conditions:
Hydrolysis: Hydrochloric acid, sodium hydroxide, and borate buffer are commonly used.
Catalysis: Phosphate and acetate buffers are used for general acid-base hydrolysis.
Major Products: The major products formed from these reactions include various hydrolyzed forms of cefoselis, depending on the pH and the specific conditions of the reaction .
Scientific Research Applications
Cefoselis hydrochloride has a broad spectrum of applications in scientific research:
Chemistry: Used in studies involving the adsorption dynamics on macroporous resin for separation and purification.
Medicine: Extensively used in clinical settings to treat respiratory and urinary tract infections.
Industry: Utilized in the pharmaceutical industry for the production of semi-synthetic cephalosporin compounds.
Mechanism of Action
Cefoselis hydrochloride exerts its effects by inhibiting bacterial cell wall synthesis. It binds to penicillin-binding proteins, which inhibits the final transpeptidation step of peptidoglycan synthesis in bacterial cell walls. This inhibition leads to bacterial cell lysis and death . The wide antibacterial spectrum of cefoselis is attributed to its resistance to hydrolysis by chromosomal β-lactamases and its rapid penetration through the bacterial cell wall .
Comparison with Similar Compounds
Cefepime: Another fourth-generation cephalosporin with a broad spectrum of activity.
Cefpirome: Known for its effectiveness against gram-negative bacteria.
Cefazolin: A first-generation cephalosporin used for treating skin and soft tissue infections.
Cefoselis hydrochloride stands out due to its unique structural features and its broad-spectrum antibacterial activity, making it a valuable compound in both clinical and research settings.
Biological Activity
Cefoselis hydrochloride is a fourth-generation cephalosporin antibiotic characterized by its broad spectrum of antibacterial activity against both Gram-positive and Gram-negative bacteria. This article delves into its biological activity, focusing on in vitro studies, pharmacokinetics, and clinical implications, supported by data tables and relevant case studies.
Overview of this compound
This compound is notable for its ability to resist hydrolysis by certain β-lactamases, which enhances its efficacy against resistant bacterial strains. Its chemical structure includes an imidazopyrazolium methyl group at the C3 position, contributing to its antibacterial properties, particularly against methicillin-resistant Staphylococcus aureus (MRSA) .
Minimum Inhibitory Concentrations (MICs)
The antibacterial activity of cefoselis has been assessed against various pathogens using the broth microdilution method. The following table summarizes the susceptibility rates of cefoselis against common bacterial pathogens:
Pathogen | Susceptibility Rate (%) | MIC (μg/mL) |
---|---|---|
Non-ESBL E. coli | 100 | < 0.5 |
Non-ESBL K. pneumoniae | 94.3 | < 0.5 |
Non-ESBL P. mirabilis | 97.0 | < 0.5 |
ESBL-producing E. coli | < 10 | > 32 |
ESBL-producing K. pneumoniae | < 10 | > 32 |
Acinetobacter baumannii | 18.7 | > 32 |
Pseudomonas aeruginosa | 73.3 | < 4 |
MRSA | 0 | - |
MSSA | 100 | < 0.5 |
Cefoselis exhibited excellent activity against non-ESBL-producing strains of Enterobacteriaceae, while showing significantly reduced efficacy against ESBL producers .
Pharmacokinetics and Blood-Brain Barrier Penetration
Cefoselis demonstrates notable penetration through the blood-brain barrier (BBB), which is critical for treating central nervous system infections. Studies have shown that cefoselis levels in brain extracellular fluid correlate with blood concentrations, indicating effective BBB penetration . This characteristic sets cefoselis apart from other β-lactams, such as cefazolin, which do not penetrate the BBB effectively.
Research indicates that cefoselis interacts with GABA receptors, inhibiting GABA-induced currents in a concentration-dependent manner. The competitive inhibition observed with cefoselis contrasts with the non-competitive inhibition seen with penicillin-G . This interaction may contribute to neurological side effects such as seizures, particularly in patients with renal impairment .
Case Study: Seizures Induced by Cefoselis
A clinical observation noted that elderly patients and those with renal failure experienced seizures when treated with cefoselis. The study indicated that while cefoselis can penetrate the BBB, excessive accumulation in the brain may lead to excitotoxicity due to altered neurotransmitter dynamics . This underscores the importance of monitoring renal function and adjusting dosages accordingly.
Stability and Degradation
Cefoselis is susceptible to degradation under various conditions. Studies have shown that it remains stable in dry heat but degrades significantly under light exposure . Understanding these stability characteristics is crucial for ensuring effective therapeutic use.
Properties
Molecular Formula |
C19H23ClN8O6S2 |
---|---|
Molecular Weight |
559.0 g/mol |
IUPAC Name |
(6R,7R)-7-[[(2Z)-2-(2-amino-1,3-thiazol-4-yl)-2-methoxyiminoacetyl]amino]-3-[[2-(2-hydroxyethyl)-3-iminopyrazol-1-yl]methyl]-8-oxo-5-thia-1-azabicyclo[4.2.0]oct-2-ene-2-carboxylic acid;hydrochloride |
InChI |
InChI=1S/C19H22N8O6S2.ClH/c1-33-24-12(10-8-35-19(21)22-10)15(29)23-13-16(30)27-14(18(31)32)9(7-34-17(13)27)6-25-3-2-11(20)26(25)4-5-28;/h2-3,8,13,17,20,28H,4-7H2,1H3,(H2,21,22)(H,23,29)(H,31,32);1H/b20-11?,24-12-;/t13-,17-;/m1./s1 |
InChI Key |
NRCXQSKOHNLPOW-ZQCAECPKSA-N |
Isomeric SMILES |
CO/N=C(/C1=CSC(=N1)N)\C(=O)N[C@H]2[C@@H]3N(C2=O)C(=C(CS3)CN4C=CC(=N)N4CCO)C(=O)O.Cl |
Canonical SMILES |
CON=C(C1=CSC(=N1)N)C(=O)NC2C3N(C2=O)C(=C(CS3)CN4C=CC(=N)N4CCO)C(=O)O.Cl |
Origin of Product |
United States |
Disclaimer and Information on In-Vitro Research Products
Please be aware that all articles and product information presented on BenchChem are intended solely for informational purposes. The products available for purchase on BenchChem are specifically designed for in-vitro studies, which are conducted outside of living organisms. In-vitro studies, derived from the Latin term "in glass," involve experiments performed in controlled laboratory settings using cells or tissues. It is important to note that these products are not categorized as medicines or drugs, and they have not received approval from the FDA for the prevention, treatment, or cure of any medical condition, ailment, or disease. We must emphasize that any form of bodily introduction of these products into humans or animals is strictly prohibited by law. It is essential to adhere to these guidelines to ensure compliance with legal and ethical standards in research and experimentation.