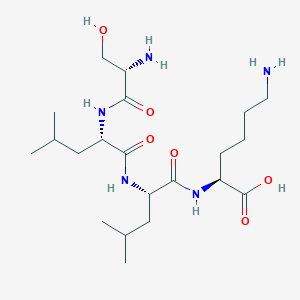
SLLK, Control Peptide for TSP1 Inhibitor
- Click on QUICK INQUIRY to receive a quote from our team of experts.
- With the quality product at a COMPETITIVE price, you can focus more on your research.
Overview
Description
SLLK (Serine-Leucine-Leucine-Lysine) is a tetrapeptide designed as a scrambled control for the thrombospondin-1 (TSP1) inhibitor LSKL (Leucine-Serine-Lysine-Leucine). TSP1 is a multifunctional glycoprotein involved in angiogenesis, TGF-β activation, and extracellular matrix remodeling. LSKL inhibits TSP1-dependent TGF-β activation by binding to TSP1's type 1 repeats, thereby blocking downstream signaling. SLLK, with a reversed amino acid sequence, serves as a critical negative control to validate the specificity of LSKL in experimental models .
Preparation Methods
Synthetic Routes and Reaction Conditions
SLLK is synthesized using solid-phase peptide synthesis (SPPS), a common method for producing peptides. The process involves the sequential addition of amino acids to a growing peptide chain anchored to a solid resin. The amino acids are protected by specific groups to prevent unwanted reactions. The synthesis typically follows these steps:
Resin Loading: The first amino acid is attached to the resin.
Deprotection: The protecting group is removed from the amino acid.
Coupling: The next amino acid is added to the chain.
Repetition: Steps 2 and 3 are repeated until the desired peptide sequence is obtained.
Cleavage: The peptide is cleaved from the resin and deprotected to yield the final product.
Industrial Production Methods
Industrial production of SLLK follows similar principles as laboratory synthesis but on a larger scale. Automated peptide synthesizers are often used to increase efficiency and consistency. The process involves rigorous quality control measures to ensure the purity and integrity of the peptide.
Chemical Reactions Analysis
Types of Reactions
SLLK undergoes various chemical reactions, including:
Oxidation: Involves the addition of oxygen or the removal of hydrogen.
Reduction: Involves the addition of hydrogen or the removal of oxygen.
Substitution: Involves the replacement of one functional group with another.
Common Reagents and Conditions
Oxidation: Common oxidizing agents include hydrogen peroxide and potassium permanganate.
Reduction: Common reducing agents include sodium borohydride and lithium aluminum hydride.
Substitution: Common reagents include alkyl halides and nucleophiles.
Major Products Formed
The major products formed from these reactions depend on the specific conditions and reagents used. For example, oxidation may yield sulfoxides or sulfones, while reduction may yield alcohols or amines.
Scientific Research Applications
Role of SLLK as a Control Peptide
SLLK is specifically designed to serve as a control for the LSKL peptide, which acts as an antagonist to TSP-1. The primary function of SLLK is to provide a baseline for experimental comparisons, allowing researchers to distinguish between the effects of thrombospondin-1 inhibition and other variables. By using SLLK alongside LSKL, researchers can ascertain that observed biological effects are indeed due to TSP-1 inhibition rather than confounding factors.
Fibrosis and Wound Healing
SLLK has been utilized in studies exploring the role of TSP-1 in fibrotic diseases. By providing a control against which the effects of LSKL can be measured, researchers have been able to demonstrate how inhibiting TSP-1 can reduce fibrosis and enhance wound healing processes. For example, studies have shown that LSKL treatment leads to significant reductions in fibrosis markers in various animal models .
Cancer Progression
In cancer research, SLLK has been instrumental in elucidating the role of TSP-1 in tumor microenvironments. By using SLLK as a control, researchers have been able to investigate how TSP-1 influences tumor growth and metastasis. Findings suggest that inhibiting TSP-1 can alter tumor progression dynamics, making it a potential target for therapeutic strategies .
Ocular Health
Recent studies have indicated that TSP-1 plays a role in regulating intraocular pressure and may be involved in conditions such as glaucoma. The use of SLLK allows researchers to assess the specific contributions of TSP-1 inhibition on ocular health outcomes without interference from other peptides .
Case Studies
Study | Objective | Findings | Significance |
---|---|---|---|
Belmadani et al., 2007 | Evaluate LSKL as a TSP-1 antagonist | LSKL effectively blocks TGF-β activation by inhibiting TSP-1 binding | Established foundation for using SLLK as a control |
PMC7294719 | Investigate TSP-1's role in fibrosis | Demonstrated reduced fibrosis with LSKL treatment compared to SLLK controls | Highlighted therapeutic potential of targeting TSP-1 |
PMC2998110 | Assess impact on VEGF signaling | Found that TSP-1 inhibits VEGF signaling pathways | Provided insights into angiogenesis regulation |
Mechanism of Action
SLLK exerts its effects by acting as a control peptide for LSKL, a TSP-1 inhibitor. TSP-1 is known to activate transforming growth factor-beta (TGF-β), a cytokine involved in various cellular processes. By inhibiting TSP-1, LSKL reduces TGF-β activity, leading to decreased cell proliferation and migration . SLLK, as a control peptide, helps researchers understand the specific effects of TSP-1 inhibition by providing a baseline for comparison.
Comparison with Similar Compounds
Key Characteristics of SLLK :
- Sequence : SLLK (H₂N-Ser-Leu-Leu-Lys-NH₂) vs. LSKL (H₂N-Leu-Ser-Lys-Leu-NH₂).
- Molecular Weight: 459.58 Da (non-TFA salt) or 573.60 Da (TFA salt) .
- CAS Number : 464924-27-4 .
- Solubility : Soluble in water (up to 125 mg/mL with sonication) and DMSO .
- Storage : Stable at -20°C (powder, 3 years) or -80°C (solution, 6 months) .
Structural and Functional Comparison: SLLK vs. LSKL
Mechanistic Insights :
- The scrambled sequence of SLLK disrupts the LSKL motif required for TSP1 binding. The positioning of lysine (K) in LSKL enables electrostatic interactions with TSP1, which are absent in SLLK .
In Vivo Efficacy: SLLK vs. LSKL
*p < 0.001 vs. SLLK
Comparison with Other Control Peptides
While SLLK is the primary control for LSKL, other scrambled peptides (e.g., GRGDSPK) or siRNA controls (e.g., non-targeting siRNA) are used in TSP1 studies. However, SLLK is uniquely validated for TSP1 research due to:
- Sequence Specificity : Direct scrambling of LSKL ensures structural comparability .
- Broad Applicability : Used in diverse models, including diabetic nephropathy , ocular hypertension , and neural stem cell differentiation .
Limitations :
- Minor Batch Variability: Peptide purity (95–98%) may affect reproducibility .
Biological Activity
The SLLK peptide, a control peptide for the LSKL sequence derived from the latency-associated peptide of transforming growth factor-beta (TGF-β), plays a significant role in the study of thrombospondin-1 (TSP1) inhibition. This article delves into the biological activity of SLLK, focusing on its role in modulating TGF-β activity, particularly in various disease models.
Overview of Thrombospondin-1 and TGF-β Interaction
Thrombospondin-1 is a matricellular protein that significantly influences cell functions such as adhesion, migration, and angiogenesis. It is known to activate latent TGF-β, which is crucial in various pathological conditions, including cancer and fibrosis. The interaction between TSP1 and TGF-β is mediated by specific sequences within these proteins, where the LSKL sequence acts as a competitive antagonist to inhibit this interaction.
Inhibition of TGF-β Activation
Research has demonstrated that the LSKL peptide effectively inhibits TSP1-mediated activation of latent TGF-β. For instance, in mouse models of multiple myeloma, administration of LSKL resulted in decreased tumor burden and reduced levels of IL-6 and osteolytic bone disease markers . The SLLK control peptide does not exhibit these inhibitory effects, making it a critical tool for comparative studies.
Renal Disease Models
In diabetic nephropathy models, the LSKL peptide showed significant therapeutic potential. Akita mice treated with LSKL exhibited reduced urinary TGF-β activity and improved renal function markers such as nephrin expression. In contrast, treatment with the SLLK control peptide did not yield similar benefits . This highlights the specificity of LSKL in targeting excessive TGF-β activity without affecting basal levels.
Study 1: Myeloma Progression
A study investigated the effects of antagonizing the TSP1-TGF-β pathway using LSKL in myeloma models. Results indicated that LSKL treatment significantly reduced myeloma progression by blocking TSP1's activation of TGF-β. This was evidenced by lower levels of active TGF-β and improved bone health markers .
Study 2: Diabetic Nephropathy
In another study focusing on diabetic nephropathy, Akita mice treated with LSKL showed marked improvements in kidney function compared to those treated with SLLK. Notably, proteinuria levels decreased significantly in the LSKL group, indicating better renal health . The study concluded that selective targeting of TGF-β activation through TSP1 inhibition could be a promising therapeutic strategy.
Data Table: Comparative Effects of LSKL vs. SLLK
Parameter | LSKL Treatment | SLLK Treatment |
---|---|---|
Active TGF-β Levels (pg/mL) | 0.10 ± 0.01 | 0.20 ± 0.02 |
Nephrin Expression (Fold Change) | >2-fold increase | No significant change |
Proteinuria Improvement | Significant reduction | No improvement |
Tumor Burden | Significantly reduced | Not applicable |
Q & A
Basic Research Questions
Q. How should SLLK be integrated into experimental designs as a control peptide for TSP1 inhibition studies?
SLLK is a scrambled peptide (sequence: Ser-Leu-Leu-Lys) used to validate the specificity of LSKL, the active TSP1 inhibitor. In murine models, SLLK serves as a negative control in dose-response experiments. For example, in diabetic nephropathy studies, Akita mice treated with 30 mg/kg LSKL showed a twofold increase in nephrin expression compared to SLLK-treated or saline controls . Key design elements include:
- Dosage parity : Administer SLLK at the same concentration as LSKL (e.g., 5–30 mg/kg).
- Endpoint validation : Measure TGF-β1 plasma levels (via ELISA) and mRNA/protein expression in target tissues (e.g., renal lysates) .
- Statistical rigor : Use ANOVA with post-hoc tests to compare SLLK vs. LSKL effects (e.g., P=0.0001 for TGF-β1 differences in plasma) .
Q. What protocols ensure SLLK stability during in vivo and in vitro applications?
SLLK’s solubility and bioactivity depend on storage conditions:
- Powder form : Store at -20°C for ≤3 years; reconstitute in sterile water or DMSO (10 mM stock) .
- Solubilized peptide : Aliquot and store at -80°C for ≤1 year to prevent freeze-thaw degradation .
- In vivo administration : Use PBS or saline as a vehicle; avoid repeated thawing by preparing single-use aliquots .
Q. How is SLLK’s inactivity confirmed in TSP1-TGF-β1 pathway studies?
Validate SLLK’s inertness using:
- TGF-β1 bioassays : Compare latent TGF-β1 activation in cell lines (e.g., HSCs) treated with LSKL (3 µM) vs. SLLK. Active LSKL reduces α-SMA and Collagen I expression by >50%, while SLLK shows no significant change .
- Oxidative stress models : In vascular smooth muscle cells (VSMCs), SLLK does not reverse H2O2 generation induced by SS ions, unlike LSKL .
Advanced Research Questions
Q. How can researchers resolve contradictions between mRNA and protein-level data in SLLK control experiments?
Discrepancies may arise from post-transcriptional regulation or assay sensitivity. For example, in aortic lysates, LSKL reduces TGF-β1 mRNA but not protein levels in some models . Mitigation strategies:
- Multi-omics validation : Pair RNA-seq with Western blotting or ELISA to quantify TGF-β1 activation.
- Time-course analysis : Assess mRNA (e.g., 24–48 hours post-treatment) vs. protein (e.g., day 42 in chronic models) .
- Pathway inhibitors : Use Smad3 inhibitors to isolate TSP1-dependent TGF-β1 effects from alternate pathways .
Q. What mechanistic insights explain SLLK’s lack of effect on TSP1-CD47 signaling in T cells?
TSP1 inhibits H2S-dependent T cell activation via CD47 binding, but SLLK lacks the critical LSKL sequence required for CD47 interaction. Experimental validation:
- Flow cytometry : CD47 knockout T cells show no response to SLLK, while wild-type cells retain baseline activity .
- ERK phosphorylation assays : SLLK does not block MEK/ERK signaling potentiated by H2S, unlike CD47-targeting peptides .
Q. How should peptide batch variability be controlled in multi-institutional studies using SLLK?
Batch-to-batch inconsistencies (e.g., salt content, solubility) may arise due to synthesis methods. Recommendations:
- QC documentation : Request mass spectrometry (MS) and HPLC purity reports (≥95%) from suppliers .
- Bioactivity normalization : Pre-test each batch in a pilot assay (e.g., TGF-β1 inhibition in VSMCs) and adjust concentrations empirically .
- Collaborative calibration : Share a reference batch across labs to standardize protocols .
Q. Methodological Considerations
Q. What in vitro models best demonstrate SLLK’s role as a control for LSKL?
- Mesangial cell culture : Treat cells with 5 µM SLLK or LSKL under amino acid starvation. LSKL, but not SLLK, restores nephrin expression by >50% .
- Fibroblast activation : In lung fibroblasts, SLLK does not alter Thy-1-mediated TGF-β activation, unlike LSKL .
Q. How can oxidative stress confound SLLK experimental outcomes, and how is this addressed?
SS ions increase H2O2 independently of TSP1, but LSKL may indirectly modulate ROS via PKG. To dissect mechanisms:
- Co-treatment with antioxidants : Use catalase (100 U/mL) to neutralize H2O2 and isolate TSP1-specific effects .
- PKG activity assays : Measure cGMP levels in VSMCs; SLLK does not rescue PKG downregulation caused by SS ions .
Properties
Molecular Formula |
C21H41N5O6 |
---|---|
Molecular Weight |
459.6 g/mol |
IUPAC Name |
(2S)-6-amino-2-[[(2S)-2-[[(2S)-2-[[(2S)-2-amino-3-hydroxypropanoyl]amino]-4-methylpentanoyl]amino]-4-methylpentanoyl]amino]hexanoic acid |
InChI |
InChI=1S/C21H41N5O6/c1-12(2)9-16(25-18(28)14(23)11-27)20(30)26-17(10-13(3)4)19(29)24-15(21(31)32)7-5-6-8-22/h12-17,27H,5-11,22-23H2,1-4H3,(H,24,29)(H,25,28)(H,26,30)(H,31,32)/t14-,15-,16-,17-/m0/s1 |
InChI Key |
HZDFWTSYGOUTRD-QAETUUGQSA-N |
Isomeric SMILES |
CC(C)C[C@@H](C(=O)N[C@@H](CCCCN)C(=O)O)NC(=O)[C@H](CC(C)C)NC(=O)[C@H](CO)N |
Canonical SMILES |
CC(C)CC(C(=O)NC(CCCCN)C(=O)O)NC(=O)C(CC(C)C)NC(=O)C(CO)N |
Origin of Product |
United States |
Disclaimer and Information on In-Vitro Research Products
Please be aware that all articles and product information presented on BenchChem are intended solely for informational purposes. The products available for purchase on BenchChem are specifically designed for in-vitro studies, which are conducted outside of living organisms. In-vitro studies, derived from the Latin term "in glass," involve experiments performed in controlled laboratory settings using cells or tissues. It is important to note that these products are not categorized as medicines or drugs, and they have not received approval from the FDA for the prevention, treatment, or cure of any medical condition, ailment, or disease. We must emphasize that any form of bodily introduction of these products into humans or animals is strictly prohibited by law. It is essential to adhere to these guidelines to ensure compliance with legal and ethical standards in research and experimentation.