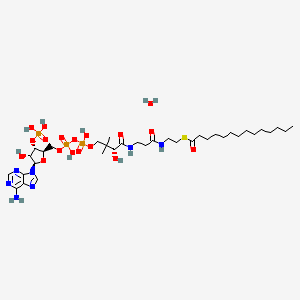
Myristoyl Coenzyme A (hydrate)
- Click on QUICK INQUIRY to receive a quote from our team of experts.
- With the quality product at a COMPETITIVE price, you can focus more on your research.
Overview
Description
Myristoyl Coenzyme A (hydrate) is a derivative of coenzyme A that contains the long-chain fatty acid myristic acid. It serves as a substrate in protein myristoylation, a process catalyzed by the enzyme N-myristoyltransferase. This process involves the transfer of the myristoyl group to the glycine residue at the amino-terminal of the protein .
Preparation Methods
Synthetic Routes and Reaction Conditions: Myristoyl Coenzyme A (hydrate) can be synthesized by combining coenzyme A with myristic acid. The reaction typically involves the activation of myristic acid to form myristoyl adenylate, which then reacts with coenzyme A to form myristoyl coenzyme A. This process is catalyzed by the enzyme acyl-CoA synthetase under specific conditions .
Industrial Production Methods: Industrial production of myristoyl coenzyme A (hydrate) involves the large-scale synthesis of coenzyme A and myristic acid, followed by their enzymatic or chemical coupling. The reaction conditions are optimized to ensure high yield and purity of the final product .
Types of Reactions:
Myristoylation: Myristoyl Coenzyme A (hydrate) undergoes myristoylation, where the myristoyl group is transferred to the N-terminal glycine residue of proteins.
De Novo Synthesis of Phosphatidylinositol: It acts as a substrate in the synthesis of phosphatidylinositol.
Common Reagents and Conditions:
Major Products:
Scientific Research Applications
Myristoyl Coenzyme A (hydrate) has a wide range of applications in scientific research:
Mechanism of Action
Myristoyl Coenzyme A (hydrate) exerts its effects through the process of myristoylation. The enzyme N-myristoyltransferase catalyzes the transfer of the myristoyl group from myristoyl coenzyme A to the N-terminal glycine residue of target proteins. This modification increases protein-protein interactions and affects the subcellular localization of the modified proteins. The myristoylation process is essential for the proper functioning of many cellular proteins and signaling pathways .
Comparison with Similar Compounds
Palmitoyl Coenzyme A: Similar to myristoyl coenzyme A, but contains the long-chain fatty acid palmitic acid.
Stearoyl Coenzyme A: Contains the long-chain fatty acid stearic acid.
Oleoyl Coenzyme A: Contains the unsaturated fatty acid oleic acid.
Uniqueness: Myristoyl Coenzyme A (hydrate) is unique due to its specific role in protein myristoylation, which is a critical post-translational modification. Unlike other fatty acyl coenzyme A derivatives, myristoyl coenzyme A specifically targets the N-terminal glycine residue of proteins, making it essential for the regulation of protein function and signaling .
Properties
Molecular Formula |
C35H64N7O18P3S |
---|---|
Molecular Weight |
995.9 g/mol |
IUPAC Name |
S-[2-[3-[[(2R)-4-[[[(2R,3S,4R,5R)-5-(6-aminopurin-9-yl)-4-hydroxy-3-phosphonooxyoxolan-2-yl]methoxy-hydroxyphosphoryl]oxy-hydroxyphosphoryl]oxy-2-hydroxy-3,3-dimethylbutanoyl]amino]propanoylamino]ethyl] tetradecanethioate;hydrate |
InChI |
InChI=1S/C35H62N7O17P3S.H2O/c1-4-5-6-7-8-9-10-11-12-13-14-15-26(44)63-19-18-37-25(43)16-17-38-33(47)30(46)35(2,3)21-56-62(53,54)59-61(51,52)55-20-24-29(58-60(48,49)50)28(45)34(57-24)42-23-41-27-31(36)39-22-40-32(27)42;/h22-24,28-30,34,45-46H,4-21H2,1-3H3,(H,37,43)(H,38,47)(H,51,52)(H,53,54)(H2,36,39,40)(H2,48,49,50);1H2/t24-,28-,29-,30+,34-;/m1./s1 |
InChI Key |
YLDPGEHCCNTXHN-NXHBBHECSA-N |
Isomeric SMILES |
CCCCCCCCCCCCCC(=O)SCCNC(=O)CCNC(=O)[C@@H](C(C)(C)COP(=O)(O)OP(=O)(O)OC[C@@H]1[C@H]([C@H]([C@@H](O1)N2C=NC3=C(N=CN=C32)N)O)OP(=O)(O)O)O.O |
Canonical SMILES |
CCCCCCCCCCCCCC(=O)SCCNC(=O)CCNC(=O)C(C(C)(C)COP(=O)(O)OP(=O)(O)OCC1C(C(C(O1)N2C=NC3=C(N=CN=C32)N)O)OP(=O)(O)O)O.O |
Origin of Product |
United States |
Disclaimer and Information on In-Vitro Research Products
Please be aware that all articles and product information presented on BenchChem are intended solely for informational purposes. The products available for purchase on BenchChem are specifically designed for in-vitro studies, which are conducted outside of living organisms. In-vitro studies, derived from the Latin term "in glass," involve experiments performed in controlled laboratory settings using cells or tissues. It is important to note that these products are not categorized as medicines or drugs, and they have not received approval from the FDA for the prevention, treatment, or cure of any medical condition, ailment, or disease. We must emphasize that any form of bodily introduction of these products into humans or animals is strictly prohibited by law. It is essential to adhere to these guidelines to ensure compliance with legal and ethical standards in research and experimentation.