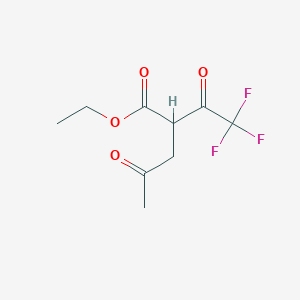
Ethyl 4-oxo-2-(2,2,2-trifluoroacetyl)pentanoate
Overview
Description
Ethyl 4-oxo-2-(2,2,2-trifluoroacetyl)pentanoate is an organic compound that belongs to the class of esters. This compound is characterized by the presence of a trifluoroacetyl group and a keto group within its structure. It is often used in organic synthesis due to its unique reactivity and the presence of the trifluoromethyl group, which imparts distinct chemical properties.
Preparation Methods
Synthetic Routes and Reaction Conditions: The synthesis of ethyl 4-oxo-2-(2,2,2-trifluoroacetyl)pentanoate typically involves the reaction of ethyl acetoacetate with trifluoroacetic anhydride. The reaction is carried out in the presence of a base, such as pyridine, to facilitate the acylation process. The general reaction scheme is as follows:
Ethyl acetoacetate+Trifluoroacetic anhydride→Ethyl 4-oxo-2-(2,2,2-trifluoroacetyl)pentanoate
Industrial Production Methods: In an industrial setting, the production of this compound may involve continuous flow reactors to ensure efficient mixing and reaction control. The use of automated systems can enhance the yield and purity of the product.
Types of Reactions:
Oxidation: this compound can undergo oxidation reactions to form various oxidized derivatives.
Reduction: The keto group in the compound can be reduced to form the corresponding alcohol.
Substitution: The trifluoromethyl group can participate in nucleophilic substitution reactions.
Common Reagents and Conditions:
Oxidation: Common oxidizing agents include potassium permanganate and chromium trioxide.
Reduction: Reducing agents such as sodium borohydride or lithium aluminum hydride are typically used.
Substitution: Nucleophiles such as amines or thiols can be used for substitution reactions.
Major Products:
Oxidation: Formation of carboxylic acids or other oxidized products.
Reduction: Formation of alcohols.
Substitution: Formation of substituted derivatives with various functional groups.
Scientific Research Applications
Ethyl 4-oxo-2-(2,2,2-trifluoroacetyl)pentanoate has a wide range of applications in scientific research:
Chemistry: Used as a building block in the synthesis of complex organic molecules.
Biology: Investigated for its potential biological activity and interactions with biomolecules.
Medicine: Explored for its potential use in drug development, particularly in the design of enzyme inhibitors.
Industry: Utilized in the production of specialty chemicals and materials with unique properties.
Mechanism of Action
The mechanism of action of ethyl 4-oxo-2-(2,2,2-trifluoroacetyl)pentanoate involves its interaction with various molecular targets. The trifluoromethyl group can enhance the compound’s lipophilicity, allowing it to interact with hydrophobic regions of proteins and enzymes. The keto group can participate in hydrogen bonding and other interactions, influencing the compound’s reactivity and binding affinity.
Comparison with Similar Compounds
Ethyl 4-oxo-2-(2,2,2-trifluoroacetyl)pentanoate can be compared with similar compounds such as:
Ethyl acetoacetate: Lacks the trifluoromethyl group, resulting in different reactivity and properties.
Mthis compound: Similar structure but with a methyl ester group instead of an ethyl ester group.
Ethyl 4-oxo-2-(2,2,2-trifluoroacetyl)butanoate: Similar structure but with a shorter carbon chain.
The presence of the trifluoromethyl group in this compound imparts unique chemical properties, making it distinct from its analogs.
Properties
IUPAC Name |
ethyl 4-oxo-2-(2,2,2-trifluoroacetyl)pentanoate | |
---|---|---|
Source | PubChem | |
URL | https://pubchem.ncbi.nlm.nih.gov | |
Description | Data deposited in or computed by PubChem | |
InChI |
InChI=1S/C9H11F3O4/c1-3-16-8(15)6(4-5(2)13)7(14)9(10,11)12/h6H,3-4H2,1-2H3 | |
Source | PubChem | |
URL | https://pubchem.ncbi.nlm.nih.gov | |
Description | Data deposited in or computed by PubChem | |
InChI Key |
FGEABMHTTHRIOZ-UHFFFAOYSA-N | |
Source | PubChem | |
URL | https://pubchem.ncbi.nlm.nih.gov | |
Description | Data deposited in or computed by PubChem | |
Canonical SMILES |
CCOC(=O)C(CC(=O)C)C(=O)C(F)(F)F | |
Source | PubChem | |
URL | https://pubchem.ncbi.nlm.nih.gov | |
Description | Data deposited in or computed by PubChem | |
Molecular Formula |
C9H11F3O4 | |
Source | PubChem | |
URL | https://pubchem.ncbi.nlm.nih.gov | |
Description | Data deposited in or computed by PubChem | |
Molecular Weight |
240.18 g/mol | |
Source | PubChem | |
URL | https://pubchem.ncbi.nlm.nih.gov | |
Description | Data deposited in or computed by PubChem | |
Synthesis routes and methods
Procedure details
Retrosynthesis Analysis
AI-Powered Synthesis Planning: Our tool employs the Template_relevance Pistachio, Template_relevance Bkms_metabolic, Template_relevance Pistachio_ringbreaker, Template_relevance Reaxys, Template_relevance Reaxys_biocatalysis model, leveraging a vast database of chemical reactions to predict feasible synthetic routes.
One-Step Synthesis Focus: Specifically designed for one-step synthesis, it provides concise and direct routes for your target compounds, streamlining the synthesis process.
Accurate Predictions: Utilizing the extensive PISTACHIO, BKMS_METABOLIC, PISTACHIO_RINGBREAKER, REAXYS, REAXYS_BIOCATALYSIS database, our tool offers high-accuracy predictions, reflecting the latest in chemical research and data.
Strategy Settings
Precursor scoring | Relevance Heuristic |
---|---|
Min. plausibility | 0.01 |
Model | Template_relevance |
Template Set | Pistachio/Bkms_metabolic/Pistachio_ringbreaker/Reaxys/Reaxys_biocatalysis |
Top-N result to add to graph | 6 |
Feasible Synthetic Routes
Disclaimer and Information on In-Vitro Research Products
Please be aware that all articles and product information presented on BenchChem are intended solely for informational purposes. The products available for purchase on BenchChem are specifically designed for in-vitro studies, which are conducted outside of living organisms. In-vitro studies, derived from the Latin term "in glass," involve experiments performed in controlled laboratory settings using cells or tissues. It is important to note that these products are not categorized as medicines or drugs, and they have not received approval from the FDA for the prevention, treatment, or cure of any medical condition, ailment, or disease. We must emphasize that any form of bodily introduction of these products into humans or animals is strictly prohibited by law. It is essential to adhere to these guidelines to ensure compliance with legal and ethical standards in research and experimentation.