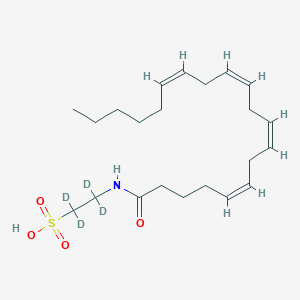
N-Arachidonoyl Taurine-d4
- Click on QUICK INQUIRY to receive a quote from our team of experts.
- With the quality product at a COMPETITIVE price, you can focus more on your research.
Overview
Description
N-Arachidonoyl Taurine-d4 is a deuterium-labeled derivative of N-Arachidonoyl Taurine. This compound is primarily used as an internal standard for the quantification of N-Arachidonoyl Taurine in various analytical applications . The incorporation of deuterium atoms into the molecule enhances its stability and allows for precise quantitation during mass spectrometry analysis .
Preparation Methods
Synthetic Routes and Reaction Conditions
The synthesis of N-Arachidonoyl Taurine-d4 involves the conjugation of deuterium-labeled arachidonic acid with taurine. The reaction typically occurs under mild conditions to preserve the integrity of the deuterium label. The process involves the activation of the carboxyl group of arachidonic acid, followed by its reaction with taurine to form the amide bond .
Industrial Production Methods
Industrial production of this compound follows similar synthetic routes but on a larger scale. The process involves the use of high-purity reagents and controlled reaction conditions to ensure the consistency and quality of the final product. The compound is then purified using chromatographic techniques to achieve the desired purity level .
Chemical Reactions Analysis
Types of Reactions
N-Arachidonoyl Taurine-d4 undergoes various chemical reactions, including oxidation, reduction, and substitution. These reactions are essential for studying the compound’s behavior and interactions in different environments .
Common Reagents and Conditions
Common reagents used in the reactions of this compound include oxidizing agents like hydrogen peroxide and reducing agents such as sodium borohydride. The reactions are typically carried out under controlled conditions to ensure the desired outcome .
Major Products Formed
The major products formed from the reactions of this compound depend on the specific reaction conditions. For example, oxidation reactions may yield hydroxylated derivatives, while reduction reactions can produce deuterium-labeled analogs with altered functional groups .
Scientific Research Applications
N-Arachidonoyl Taurine-d4 has a wide range of scientific research applications, including:
Mechanism of Action
N-Arachidonoyl Taurine-d4 exerts its effects by interacting with specific molecular targets and pathways. It is known to activate transient receptor potential vanilloid (TRPV) channels, particularly TRPV1 and TRPV4 . This activation leads to increased calcium flux in pancreatic β-cells, which in turn stimulates insulin secretion . Additionally, this compound has been shown to restore the function of mutant ion channels associated with Long QT syndrome by modulating their gating properties .
Comparison with Similar Compounds
Similar Compounds
N-Arachidonoyl Taurine: The non-deuterated form of N-Arachidonoyl Taurine-d4, used in similar applications but without the enhanced stability provided by deuterium labeling.
N-Oleoyl Taurine: Another fatty acid-taurine conjugate with similar biological activities, including inhibition of cell proliferation in cancer cells.
N-Palmitoyl Taurine: A fatty acid-taurine conjugate with distinct biological properties and applications in lipid metabolism research.
Uniqueness
N-Arachidonoyl Taurine-d4 is unique due to its deuterium labeling, which provides enhanced stability and allows for precise quantitation in analytical applications. This makes it a valuable tool in scientific research, particularly in studies involving mass spectrometry and pharmacokinetics .
Properties
Molecular Formula |
C22H37NO4S |
---|---|
Molecular Weight |
415.6 g/mol |
IUPAC Name |
1,1,2,2-tetradeuterio-2-[[(5Z,8Z,11Z,14Z)-icosa-5,8,11,14-tetraenoyl]amino]ethanesulfonic acid |
InChI |
InChI=1S/C22H37NO4S/c1-2-3-4-5-6-7-8-9-10-11-12-13-14-15-16-17-18-19-22(24)23-20-21-28(25,26)27/h6-7,9-10,12-13,15-16H,2-5,8,11,14,17-21H2,1H3,(H,23,24)(H,25,26,27)/b7-6-,10-9-,13-12-,16-15-/i20D2,21D2 |
InChI Key |
YUNYSWCRLRYOPO-KALLKTRHSA-N |
Isomeric SMILES |
[2H]C([2H])(C([2H])([2H])S(=O)(=O)O)NC(=O)CCC/C=C\C/C=C\C/C=C\C/C=C\CCCCC |
Canonical SMILES |
CCCCCC=CCC=CCC=CCC=CCCCC(=O)NCCS(=O)(=O)O |
Origin of Product |
United States |
Disclaimer and Information on In-Vitro Research Products
Please be aware that all articles and product information presented on BenchChem are intended solely for informational purposes. The products available for purchase on BenchChem are specifically designed for in-vitro studies, which are conducted outside of living organisms. In-vitro studies, derived from the Latin term "in glass," involve experiments performed in controlled laboratory settings using cells or tissues. It is important to note that these products are not categorized as medicines or drugs, and they have not received approval from the FDA for the prevention, treatment, or cure of any medical condition, ailment, or disease. We must emphasize that any form of bodily introduction of these products into humans or animals is strictly prohibited by law. It is essential to adhere to these guidelines to ensure compliance with legal and ethical standards in research and experimentation.