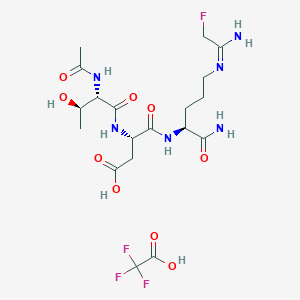
TDFA (trifluoroacetate salt)
- Click on QUICK INQUIRY to receive a quote from our team of experts.
- With the quality product at a COMPETITIVE price, you can focus more on your research.
Overview
Description
TDFA (Thr-Asp-F-amidine trifluoroacetate salt) is a selective inhibitor of peptidylarginine deiminase 4 (PAD4), an enzyme implicated in inflammatory diseases, cancer, and autoimmune disorders. Its chemical formula is C₁₇H₂₉FN₆O₇•XCF₃COOH, with a molecular weight of 448.5 g/mol . TDFA is provided as a research-grade compound, soluble in dimethylformamide (DMF, 33 mg/mL), dimethyl sulfoxide (DMSO, 33 mg/mL), ethanol (16 mg/mL), and phosphate-buffered saline (PBS, pH 7.2, 10 mg/mL). It requires storage at -20°C to maintain stability and avoid repeated freeze-thaw cycles .
Mechanistically, TDFA binds to PAD4 via interactions with residues Q346, R374, and R639, enhancing its selectivity and potency . It has demonstrated efficacy in preclinical models, such as suppressing neutrophil adhesion in vascular inflammation by inhibiting PAD4 cytoplasmic translocation and mitigating lipopolysaccharide-induced acute lung injury (ALI) in mice . TDFA is also converted into biotin-TDFA, a chemical probe for isolating PAD4 in vitro .
Preparation Methods
TDFA (trifluoroacetate salt) is synthesized through a series of chemical reactions designed to enhance its selectivity and potency. The synthetic route involves the use of specific reagents and conditions to achieve the desired product. The preparation of trifluoroacetic acid (TFA), a related compound, involves the electrofluorination of acetyl chloride or acetic anhydride, followed by hydrolysis of the resulting trifluoroacetyl fluoride . This method can be adapted for the synthesis of TDFA by incorporating additional steps to introduce the necessary functional groups.
Chemical Reactions Analysis
TDFA (trifluoroacetate salt) undergoes various chemical reactions, including oxidation, reduction, and substitution. Common reagents used in these reactions include sodium trifluoroacetate, acetic acid, and fluoride . The major products formed from these reactions depend on the specific conditions and reagents used. For example, the oxidation of TDFA can lead to the formation of citrullinated proteins, which are important in various biological processes .
Scientific Research Applications
TDFA (trifluoroacetate salt) has a wide range of scientific research applications. It is primarily used as a selective inhibitor of PAD4, which is involved in the regulation of pluripotency, stem-cell gene activation, induced pluripotent stem (iPS) cell reprogramming, and maintenance of pluripotent cells in the early mouse embryo . Additionally, TDFA is used in cancer and inflammatory disease research, as PAD4 is upregulated in these conditions . The compound’s ability to inhibit citrullination makes it valuable in studying rheumatoid arthritis onset and progression .
Mechanism of Action
TDFA (trifluoroacetate salt) exerts its effects by selectively inhibiting PAD4. By inhibiting PAD4, TDFA prevents the citrullination of proteins, thereby affecting various biological processes, including gene regulation and immune response . The molecular targets of TDFA include specific arginine residues in proteins, and the pathways involved are related to calcium-dependent enzymatic activity .
Comparison with Similar Compounds
Structural and Functional Comparison of PAD4 Inhibitors
Table 1: Key PAD4 Inhibitors and Their Properties
Key Findings :
- Salt Form Impact: TDFA and GSK121 share the trifluoroacetate counterion, which enhances solubility in organic solvents compared to hydrochloride salts like GSK199 .
- Selectivity : TDFA and GSK121 are PAD4-selective, whereas F-Amidine inhibits multiple PAD isoforms (pan-PAD) .
- Efficacy : TDFA’s potency is attributed to its unique interactions with PAD4’s catalytic site, while YW3-56 achieves PAD4 inhibition at low micromolar IC₅₀ values .
Comparison with Other Trifluoroacetate Salts
Table 2: Trifluoroacetate Salts in Research
Key Findings :
- Biological vs. Material Science Use : TDFA is tailored for enzymatic inhibition, while Tachyplesin I (a trifluoroacetate salt) serves as an antimicrobial peptide .
- Solubility : TDFA’s PBS solubility (10 mg/mL) is critical for cell-based assays, contrasting with uranium-TFA complexes that require DMSO/trifluoroacetic acid mixtures .
Comparison with Acetate Salts
For example:
Q & A
Q. Basic: What are the critical considerations for handling trifluoroacetate salts (TFA) in laboratory settings to ensure safety and prevent contamination?
Methodological Answer:
- Safety Protocols : TFA is highly corrosive; always use protective gear (gloves, goggles) and work under a fume hood to avoid inhalation or skin contact .
- Equipment : Use glass pipettes/syringes instead of plastic, as repeated plastic use can leach contaminants into TFA solutions, interfering with downstream analyses like mass spectrometry .
- Contamination Control : TFA is a common environmental contaminant due to its use in industrial processes (e.g., refrigerant degradation). Implement blank tests during analytical workflows to detect background contamination .
- Waste Disposal : Segregate TFA waste and dispose via certified hazardous waste management services to prevent environmental release .
Q. Advanced: How can researchers accurately quantify trifluoroacetate (TFA) concentrations in environmental samples, given challenges in extraction efficiency and matrix interference?
Methodological Answer:
- Internal Standards : Use high-purity, mass-labeled TFA (e.g., ¹³C-TFA) as an internal standard to correct for matrix effects and ionization efficiency variations. Avoid surrogates like PFBA, which introduce quantification errors .
- Sample Acidification : Add acid (e.g., HCl) to reduce anion competition (e.g., bicarbonate) and disrupt ion-pair formation between TFA and matrix components, improving extraction recovery .
- Matrix-Specific Optimization : Validate extraction methods (e.g., liquid-liquid extraction, solid-phase extraction) for each sample type (e.g., precipitation, surface water) to account for variability in organic content and salinity .
- Instrumentation : Employ LC-MS/MS with ion-pairing chromatography for high sensitivity (detection limits <0.1 ng/L) .
Q. Basic: What are the primary environmental sources and pathways leading to the accumulation of TFA in aquatic systems?
Methodological Answer:
- Atmospheric Deposition : TFA forms via degradation of hydrofluorocarbons (HFCs) and is globally distributed through rainfall. Concentrations in precipitation range from <0.1 ng/L to 2.4 µg/L, with higher levels near urban areas .
- Industrial Emissions : Direct discharge from pharmaceutical and chemical manufacturing sites contributes to localized contamination, e.g., riverine TFA levels up to 140 µg/L downstream of factories .
- Persistence : TFA resists biodegradation and photolysis, leading to long-term accumulation in water bodies. A 17-fold increase in TFA concentrations was observed in Chinese surface waters from 2002–2012 .
Q. Advanced: How does the use of trifluoroacetate salts in peptide synthesis impact experimental outcomes, and what mitigation strategies exist for TFA-related artifacts?
Methodological Answer:
- Protonation Artifacts : TFA is used to protonate peptides lacking basic residues (e.g., Arg, Lys), but residual TFA in final products can alter charge states and interfere with biological assays (e.g., cell toxicity studies) .
- Mitigation Strategies :
- Purification : Use reversed-phase HPLC or dialysis to remove TFA residues.
- Alternative Acids : Replace TFA with volatile alternatives (e.g., acetic acid) during synthesis where feasible.
- Quality Control : Quantify TFA content via ion chromatography or conduct spike-in recovery experiments to assess interference .
Q. Advanced: What methodologies are recommended for assessing the long-term ecological impacts of TFA, considering its persistence and bioaccumulation potential?
Methodological Answer:
- Longitudinal Monitoring : Track TFA concentrations in water, soil, and biota over decades to model accumulation trends. For example, surface water monitoring in Northern California and Alaska revealed increasing TFA levels linked to HFC use .
- Toxicity Assays : Conduct chronic exposure studies on aquatic organisms (e.g., Daphnia magna) to establish no-observed-effect concentrations (NOECs). Current data gaps exist for ultra-short-chain PFAAs beyond TFA .
- Degradation Studies : Investigate advanced oxidation processes (e.g., UV/H₂O₂) for TFA breakdown, though efficacy is limited due to its stable C-F bonds .
Q. Basic: What are the current gaps in understanding the toxicological profile of TFA, and what research approaches are needed to address them?
Methodological Answer:
- Data Gaps : Limited studies on chronic toxicity, endocrine disruption, and transgenerational effects in mammals. Human exposure risks via drinking water remain unquantified .
- Research Priorities :
Q. Advanced: How can isotopic labeling techniques improve the traceability and quantification of TFA in complex biological matrices?
Methodological Answer:
- Stable Isotope Tracers : Synthesize ¹³C- or ¹⁵N-labeled TFA to differentiate endogenous vs. exogenous sources in tissues or biofluids. This is critical for pharmacokinetic studies .
- Mass Spectrometry Workflows : Use isotope dilution analysis (IDA) with LC-HRMS to achieve precision (±5%) in serum/plasma samples. Correct for matrix suppression via standard addition curves .
- Environmental Forensics : Apply dual-isotope (δ¹³C, δ²H) analysis to trace TFA sources (e.g., industrial vs. atmospheric) in groundwater .
Properties
Molecular Formula |
C19H30F4N6O9 |
---|---|
Molecular Weight |
562.5 g/mol |
IUPAC Name |
(3S)-3-[[(2S,3R)-2-acetamido-3-hydroxybutanoyl]amino]-4-[[(2S)-1-amino-5-[(1-amino-2-fluoroethylidene)amino]-1-oxopentan-2-yl]amino]-4-oxobutanoic acid;2,2,2-trifluoroacetic acid |
InChI |
InChI=1S/C17H29FN6O7.C2HF3O2/c1-8(25)14(22-9(2)26)17(31)24-11(6-13(27)28)16(30)23-10(15(20)29)4-3-5-21-12(19)7-18;3-2(4,5)1(6)7/h8,10-11,14,25H,3-7H2,1-2H3,(H2,19,21)(H2,20,29)(H,22,26)(H,23,30)(H,24,31)(H,27,28);(H,6,7)/t8-,10+,11+,14+;/m1./s1 |
InChI Key |
PHGHISQRSBKXGU-UUUUJWPZSA-N |
Isomeric SMILES |
C[C@H]([C@@H](C(=O)N[C@@H](CC(=O)O)C(=O)N[C@@H](CCCN=C(CF)N)C(=O)N)NC(=O)C)O.C(=O)(C(F)(F)F)O |
Canonical SMILES |
CC(C(C(=O)NC(CC(=O)O)C(=O)NC(CCCN=C(CF)N)C(=O)N)NC(=O)C)O.C(=O)(C(F)(F)F)O |
Origin of Product |
United States |
Disclaimer and Information on In-Vitro Research Products
Please be aware that all articles and product information presented on BenchChem are intended solely for informational purposes. The products available for purchase on BenchChem are specifically designed for in-vitro studies, which are conducted outside of living organisms. In-vitro studies, derived from the Latin term "in glass," involve experiments performed in controlled laboratory settings using cells or tissues. It is important to note that these products are not categorized as medicines or drugs, and they have not received approval from the FDA for the prevention, treatment, or cure of any medical condition, ailment, or disease. We must emphasize that any form of bodily introduction of these products into humans or animals is strictly prohibited by law. It is essential to adhere to these guidelines to ensure compliance with legal and ethical standards in research and experimentation.