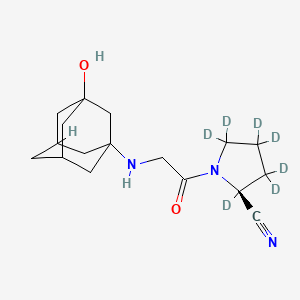
Vildagliptin-d7
- Click on QUICK INQUIRY to receive a quote from our team of experts.
- With the quality product at a COMPETITIVE price, you can focus more on your research.
Overview
Description
Vildagliptin-d7 is a deuterated form of Vildagliptin, an oral anti-hyperglycemic agent used in the management of type 2 diabetes mellitus. Vildagliptin belongs to the dipeptidyl peptidase-4 (DPP-4) inhibitor class of drugs, which work by inhibiting the enzyme DPP-4, thereby increasing the levels of incretin hormones that regulate blood glucose levels .
Preparation Methods
Synthetic Routes and Reaction Conditions
The synthesis of Vildagliptin-d7 involves the incorporation of deuterium atoms into the Vildagliptin molecule. One common method involves the use of deuterated reagents in the synthesis process. For example, L-proline can be reacted with chloroacetyl chloride in the presence of deuterated solvents to introduce deuterium atoms into the final product .
Industrial Production Methods
Industrial production of this compound follows similar synthetic routes as the laboratory synthesis but on a larger scale. The process involves the use of high-purity deuterated reagents and solvents to ensure the incorporation of deuterium atoms. The reaction conditions are optimized to maximize yield and purity, and the final product is purified using techniques such as high-performance liquid chromatography (HPLC) .
Chemical Reactions Analysis
Types of Reactions
Vildagliptin-d7 undergoes various chemical reactions, including:
Oxidation: this compound can be oxidized to form corresponding oxides.
Reduction: Reduction reactions can convert this compound to its reduced forms.
Substitution: Substitution reactions can replace specific atoms or groups within the molecule.
Common Reagents and Conditions
Oxidation: Common oxidizing agents include hydrogen peroxide and potassium permanganate.
Reduction: Reducing agents such as sodium borohydride and lithium aluminum hydride are used.
Substitution: Reagents like halogens and nucleophiles are used under various conditions.
Major Products Formed
The major products formed from these reactions depend on the specific reagents and conditions used. For example, oxidation may yield oxides, while reduction may produce alcohols or amines .
Scientific Research Applications
Vildagliptin-d7 has several scientific research applications, including:
Chemistry: Used as a reference standard in analytical chemistry to study the behavior of deuterated compounds.
Biology: Employed in metabolic studies to understand the pharmacokinetics and pharmacodynamics of deuterated drugs.
Medicine: Investigated for its potential therapeutic benefits in managing type 2 diabetes mellitus.
Industry: Used in the development of new pharmaceutical formulations and drug delivery systems
Mechanism of Action
Vildagliptin-d7 exerts its effects by inhibiting the enzyme dipeptidyl peptidase-4 (DPP-4). This inhibition prevents the degradation of incretin hormones, such as glucagon-like peptide-1 (GLP-1) and glucose-dependent insulinotropic polypeptide (GIP). Elevated levels of these hormones enhance insulin secretion and suppress glucagon release, thereby improving glycemic control .
Comparison with Similar Compounds
Similar Compounds
Sitagliptin: Another DPP-4 inhibitor used in the management of type 2 diabetes mellitus.
Saxagliptin: A DPP-4 inhibitor with a similar mechanism of action.
Linagliptin: Known for its high selectivity for DPP-4.
Uniqueness of Vildagliptin-d7
This compound is unique due to the incorporation of deuterium atoms, which can enhance the metabolic stability and pharmacokinetic properties of the compound. This can lead to improved efficacy and reduced side effects compared to non-deuterated analogs .
Biological Activity
Vildagliptin-d7 is a deuterium-labeled derivative of vildagliptin, a selective dipeptidyl peptidase IV (DPP-IV) inhibitor. This compound is primarily utilized in research to investigate the pharmacokinetics and biological activity of vildagliptin. The incorporation of deuterium helps in tracing the compound during metabolic studies, enhancing the understanding of its behavior in biological systems.
Property | Value |
---|---|
CAS Number | 1133208-42-0 |
Molecular Formula | C17H18D7N3O2 |
Molecular Weight | 310.44 g/mol |
Density | 1.3 ± 0.1 g/cm³ |
Boiling Point | 531.3 ± 50.0 °C at 760 mmHg |
Melting Point | N/A |
Flash Point | 275.1 ± 30.1 °C |
This compound functions by inhibiting DPP-IV, an enzyme responsible for the degradation of incretin hormones such as GLP-1 (glucagon-like peptide-1) and GIP (glucose-dependent insulinotropic polypeptide). By preventing the breakdown of these hormones, this compound enhances insulin secretion in response to meals and decreases glucagon levels, leading to improved glycemic control.
Efficacy in Clinical Studies
Research has demonstrated that vildagliptin significantly improves glycemic control in patients with type 2 diabetes mellitus (T2DM). A notable study showed that after 24 weeks, patients treated with vildagliptin experienced a reduction in HbA1c levels by an average of -1.01%, compared to -0.25% in the placebo group (p < 0.001) . Additionally, vildagliptin has been shown to be effective as an add-on therapy to metformin, providing significant reductions in fasting plasma glucose levels .
Case Studies
Case Study 1: Efficacy and Safety Assessment
In a randomized controlled trial involving patients inadequately controlled with metformin and glimepiride, those treated with vildagliptin achieved a greater reduction in HbA1c levels compared to placebo . The trial included a sample size of 246 patients, ensuring robust statistical power for the findings.
Case Study 2: Pharmacokinetic Analysis
A study optimized methods for analyzing vildagliptin levels in human plasma, demonstrating high accuracy and precision (97.30%-104.15%) using electrospray ionization mass spectrometry . This advancement allows for better understanding of vildagliptin's pharmacokinetic profile and its deuterated form, this compound.
Pharmacokinetics
The pharmacokinetic properties of this compound have been explored through various studies, revealing that deuteration may influence the drug's metabolic stability and half-life. The incorporation of deuterium is expected to slow down metabolic processes, potentially leading to prolonged action within the body .
Comparative Studies
Research indicates that vildagliptin exhibits similar efficacy when compared to other DPP-IV inhibitors but with a significantly lower risk of hypoglycemia and weight gain . The combination of vildagliptin with metformin has been particularly noted for its weight-neutral profile and safety in clinical practice settings.
Properties
IUPAC Name |
(2S)-2,3,3,4,4,5,5-heptadeuterio-1-[2-[(3-hydroxy-1-adamantyl)amino]acetyl]pyrrolidine-2-carbonitrile |
Source
|
---|---|---|
Source | PubChem | |
URL | https://pubchem.ncbi.nlm.nih.gov | |
Description | Data deposited in or computed by PubChem | |
InChI |
InChI=1S/C17H25N3O2/c18-9-14-2-1-3-20(14)15(21)10-19-16-5-12-4-13(6-16)8-17(22,7-12)11-16/h12-14,19,22H,1-8,10-11H2/t12?,13?,14-,16?,17?/m0/s1/i1D2,2D2,3D2,14D |
Source
|
Source | PubChem | |
URL | https://pubchem.ncbi.nlm.nih.gov | |
Description | Data deposited in or computed by PubChem | |
InChI Key |
SYOKIDBDQMKNDQ-KRANLNKXSA-N |
Source
|
Source | PubChem | |
URL | https://pubchem.ncbi.nlm.nih.gov | |
Description | Data deposited in or computed by PubChem | |
Canonical SMILES |
C1CC(N(C1)C(=O)CNC23CC4CC(C2)CC(C4)(C3)O)C#N |
Source
|
Source | PubChem | |
URL | https://pubchem.ncbi.nlm.nih.gov | |
Description | Data deposited in or computed by PubChem | |
Isomeric SMILES |
[2H][C@]1(C(C(C(N1C(=O)CNC23CC4CC(C2)CC(C4)(C3)O)([2H])[2H])([2H])[2H])([2H])[2H])C#N |
Source
|
Source | PubChem | |
URL | https://pubchem.ncbi.nlm.nih.gov | |
Description | Data deposited in or computed by PubChem | |
Molecular Formula |
C17H25N3O2 |
Source
|
Source | PubChem | |
URL | https://pubchem.ncbi.nlm.nih.gov | |
Description | Data deposited in or computed by PubChem | |
Molecular Weight |
310.44 g/mol |
Source
|
Source | PubChem | |
URL | https://pubchem.ncbi.nlm.nih.gov | |
Description | Data deposited in or computed by PubChem | |
Disclaimer and Information on In-Vitro Research Products
Please be aware that all articles and product information presented on BenchChem are intended solely for informational purposes. The products available for purchase on BenchChem are specifically designed for in-vitro studies, which are conducted outside of living organisms. In-vitro studies, derived from the Latin term "in glass," involve experiments performed in controlled laboratory settings using cells or tissues. It is important to note that these products are not categorized as medicines or drugs, and they have not received approval from the FDA for the prevention, treatment, or cure of any medical condition, ailment, or disease. We must emphasize that any form of bodily introduction of these products into humans or animals is strictly prohibited by law. It is essential to adhere to these guidelines to ensure compliance with legal and ethical standards in research and experimentation.