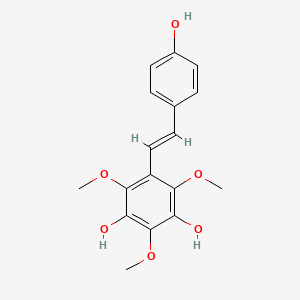
trans-Trismethoxy resveratrol
- Click on QUICK INQUIRY to receive a quote from our team of experts.
- With the quality product at a COMPETITIVE price, you can focus more on your research.
Overview
Description
trans-Trismethoxy Resveratrol: is a naturally occurring organic compound found in plants such as Pterobolium hexapetallum and Virola cuspidata . It is a methyl analog of resveratrol, derived by the addition of three methyl esters. This modification enhances its stability and bioavailability compared to resveratrol . This compound exhibits various biological properties, including protection against oxidative stress-induced DNA damage, anti-inflammatory effects, and anti-cancer activities .
Preparation Methods
Synthetic Routes and Reaction Conditions: : trans-Trismethoxy Resveratrol can be synthesized from resveratrol by converting the three active hydroxy groups into methyl esters . This process involves methylation reactions under specific conditions to achieve the desired product. The synthetic route typically includes the use of methylating agents such as dimethyl sulfate or methyl iodide in the presence of a base like potassium carbonate .
Industrial Production Methods: : Industrial production of this compound involves large-scale methylation of resveratrol using optimized reaction conditions to ensure high yield and purity . The process may include steps such as purification through column chromatography and recrystallization to obtain the final product .
Chemical Reactions Analysis
Types of Reactions: : trans-Trismethoxy Resveratrol undergoes various chemical reactions, including oxidation, reduction, and substitution .
Common Reagents and Conditions
Reduction: Reduction reactions can be carried out using reagents such as sodium borohydride or lithium aluminum hydride.
Substitution: Substitution reactions may involve nucleophilic or electrophilic reagents, depending on the desired product.
Major Products: : The major products formed from these reactions include various derivatives of this compound with modified functional groups, which can exhibit different biological activities .
Scientific Research Applications
Mechanism of Action
The mechanism of action of trans-Trismethoxy Resveratrol involves several molecular targets and pathways . It inhibits cancer cell growth by downregulating stearoyl-CoA desaturase genes, leading to a decrease in the desaturation index of fatty acids . Additionally, it exerts anti-inflammatory effects by inhibiting the activation of MAPK and NF-κB signaling pathways . The compound also protects against oxidative stress-induced DNA damage by scavenging reactive oxygen species .
Comparison with Similar Compounds
Similar Compounds
Resveratrol: The parent compound of trans-Trismethoxy Resveratrol, known for its antioxidant and anti-inflammatory properties.
3,4’,5-Trimethoxy-trans-stilbene: Another methoxy derivative of resveratrol with anti-inflammatory effects.
3,3’,4,5’-Tetramethoxy-trans-stilbene: A derivative that suppresses inflammation through inactivation of MAPK and NF-κB pathways.
Uniqueness: : trans-Trismethoxy Resveratrol is unique due to its enhanced stability and bioavailability compared to resveratrol . The addition of three methyl esters increases its resistance to metabolic degradation, allowing it to exhibit stronger biological effects .
Properties
Molecular Formula |
C17H18O6 |
---|---|
Molecular Weight |
318.32 g/mol |
IUPAC Name |
5-[(E)-2-(4-hydroxyphenyl)ethenyl]-2,4,6-trimethoxybenzene-1,3-diol |
InChI |
InChI=1S/C17H18O6/c1-21-15-12(9-6-10-4-7-11(18)8-5-10)16(22-2)14(20)17(23-3)13(15)19/h4-9,18-20H,1-3H3/b9-6+ |
InChI Key |
YVJOQDFSMTXJCV-RMKNXTFCSA-N |
Isomeric SMILES |
COC1=C(C(=C(C(=C1O)OC)O)OC)/C=C/C2=CC=C(C=C2)O |
Canonical SMILES |
COC1=C(C(=C(C(=C1O)OC)O)OC)C=CC2=CC=C(C=C2)O |
Origin of Product |
United States |
Disclaimer and Information on In-Vitro Research Products
Please be aware that all articles and product information presented on BenchChem are intended solely for informational purposes. The products available for purchase on BenchChem are specifically designed for in-vitro studies, which are conducted outside of living organisms. In-vitro studies, derived from the Latin term "in glass," involve experiments performed in controlled laboratory settings using cells or tissues. It is important to note that these products are not categorized as medicines or drugs, and they have not received approval from the FDA for the prevention, treatment, or cure of any medical condition, ailment, or disease. We must emphasize that any form of bodily introduction of these products into humans or animals is strictly prohibited by law. It is essential to adhere to these guidelines to ensure compliance with legal and ethical standards in research and experimentation.