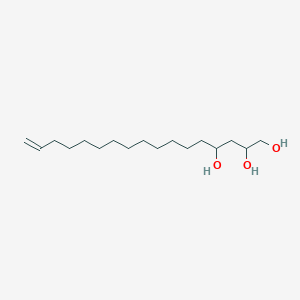
Avocadene
Overview
Description
Avocadene is a polyhydroxylated fatty alcohol derived from the avocado (Persea americana). This compound is known for its unique structure and significant biological activities, including anti-cancer, anti-inflammatory, and anti-microbial properties . This compound is often studied alongside avocadyne, another polyhydroxylated fatty alcohol, due to their similar structures and complementary biological effects .
Preparation Methods
Synthetic Routes and Reaction Conditions
Avocadene can be synthesized from avocado seed extracts. The process involves the extraction of avocado seeds using ethyl acetate, followed by purification to obtain a fraction pure in this compound and avocadyne at a 1:1 molar ratio . The synthetic route typically includes:
Extraction: Avocado seeds are ground and extracted with ethyl acetate.
Purification: The extract is purified using column chromatography to isolate this compound.
Industrial Production Methods
Industrial production of this compound involves large-scale extraction and purification processes. The seeds are processed in bulk, and the extraction is carried out using industrial-grade solvents. The purification process is optimized for high yield and purity, often involving advanced chromatographic techniques .
Chemical Reactions Analysis
Types of Reactions
Avocadene undergoes various chemical reactions, including:
Oxidation: this compound can be oxidized to form corresponding ketones or aldehydes.
Reduction: Reduction of this compound can yield saturated alcohols.
Substitution: This compound can undergo nucleophilic substitution reactions, particularly at the hydroxyl groups.
Common Reagents and Conditions
Oxidation: Common oxidizing agents include potassium permanganate and chromium trioxide.
Reduction: Reducing agents such as lithium aluminum hydride or sodium borohydride are used.
Substitution: Nucleophiles like halides or amines can be used under basic conditions.
Major Products
Oxidation: Produces ketones or aldehydes.
Reduction: Produces saturated alcohols.
Substitution: Produces substituted derivatives with various functional groups.
Scientific Research Applications
Avocadene has a wide range of scientific research applications:
Chemistry: Used as a precursor in the synthesis of complex molecules.
Biology: Studied for its role in cellular metabolism and signaling pathways.
Mechanism of Action
Avocadene exerts its effects primarily by targeting mitochondria and inhibiting fatty acid oxidation. This inhibition leads to selective apoptosis in leukemia cells and reversal of insulin resistance in diet-induced obesity . The molecular targets include enzymes involved in the fatty acid oxidation pathway, and the suppression of this pathway is critical for its biological activity .
Comparison with Similar Compounds
Avocadene is often compared with other polyhydroxylated fatty alcohols such as avocadyne and avocadenol-A. These compounds share similar structures but differ in their specific biological activities:
Avocadyne: Known for its anti-leukemia and anti-viral properties.
Avocadenol-A: Exhibits significant larvicidal activity against mosquito species.
The uniqueness of this compound lies in its ability to form a eutectic mixture with avocadyne, enhancing its incorporation into drug delivery systems and improving the bioavailability of therapeutic agents .
Properties
IUPAC Name |
heptadec-16-ene-1,2,4-triol | |
---|---|---|
Source | PubChem | |
URL | https://pubchem.ncbi.nlm.nih.gov | |
Description | Data deposited in or computed by PubChem | |
InChI |
InChI=1S/C17H34O3/c1-2-3-4-5-6-7-8-9-10-11-12-13-16(19)14-17(20)15-18/h2,16-20H,1,3-15H2 | |
Source | PubChem | |
URL | https://pubchem.ncbi.nlm.nih.gov | |
Description | Data deposited in or computed by PubChem | |
InChI Key |
DFEHQWFIOMAGBM-UHFFFAOYSA-N | |
Source | PubChem | |
URL | https://pubchem.ncbi.nlm.nih.gov | |
Description | Data deposited in or computed by PubChem | |
Canonical SMILES |
C=CCCCCCCCCCCCC(CC(CO)O)O | |
Source | PubChem | |
URL | https://pubchem.ncbi.nlm.nih.gov | |
Description | Data deposited in or computed by PubChem | |
Molecular Formula |
C17H34O3 | |
Source | PubChem | |
URL | https://pubchem.ncbi.nlm.nih.gov | |
Description | Data deposited in or computed by PubChem | |
DSSTOX Substance ID |
DTXSID001003849 | |
Record name | Heptadec-16-ene-1,2,4-triol | |
Source | EPA DSSTox | |
URL | https://comptox.epa.gov/dashboard/DTXSID001003849 | |
Description | DSSTox provides a high quality public chemistry resource for supporting improved predictive toxicology. | |
Molecular Weight |
286.4 g/mol | |
Source | PubChem | |
URL | https://pubchem.ncbi.nlm.nih.gov | |
Description | Data deposited in or computed by PubChem | |
Physical Description |
Solid | |
Record name | Avocadene | |
Source | Human Metabolome Database (HMDB) | |
URL | http://www.hmdb.ca/metabolites/HMDB0031042 | |
Description | The Human Metabolome Database (HMDB) is a freely available electronic database containing detailed information about small molecule metabolites found in the human body. | |
Explanation | HMDB is offered to the public as a freely available resource. Use and re-distribution of the data, in whole or in part, for commercial purposes requires explicit permission of the authors and explicit acknowledgment of the source material (HMDB) and the original publication (see the HMDB citing page). We ask that users who download significant portions of the database cite the HMDB paper in any resulting publications. | |
CAS No. |
83797-45-9 | |
Record name | 16-Heptadecene-1,2,4-triol | |
Source | CAS Common Chemistry | |
URL | https://commonchemistry.cas.org/detail?cas_rn=83797-45-9 | |
Description | CAS Common Chemistry is an open community resource for accessing chemical information. Nearly 500,000 chemical substances from CAS REGISTRY cover areas of community interest, including common and frequently regulated chemicals, and those relevant to high school and undergraduate chemistry classes. This chemical information, curated by our expert scientists, is provided in alignment with our mission as a division of the American Chemical Society. | |
Explanation | The data from CAS Common Chemistry is provided under a CC-BY-NC 4.0 license, unless otherwise stated. | |
Record name | 16-Heptadecene-1,2,4-triol | |
Source | ChemIDplus | |
URL | https://pubchem.ncbi.nlm.nih.gov/substance/?source=chemidplus&sourceid=0083797459 | |
Description | ChemIDplus is a free, web search system that provides access to the structure and nomenclature authority files used for the identification of chemical substances cited in National Library of Medicine (NLM) databases, including the TOXNET system. | |
Record name | Heptadec-16-ene-1,2,4-triol | |
Source | EPA DSSTox | |
URL | https://comptox.epa.gov/dashboard/DTXSID001003849 | |
Description | DSSTox provides a high quality public chemistry resource for supporting improved predictive toxicology. | |
Record name | Avocadene | |
Source | Human Metabolome Database (HMDB) | |
URL | http://www.hmdb.ca/metabolites/HMDB0031042 | |
Description | The Human Metabolome Database (HMDB) is a freely available electronic database containing detailed information about small molecule metabolites found in the human body. | |
Explanation | HMDB is offered to the public as a freely available resource. Use and re-distribution of the data, in whole or in part, for commercial purposes requires explicit permission of the authors and explicit acknowledgment of the source material (HMDB) and the original publication (see the HMDB citing page). We ask that users who download significant portions of the database cite the HMDB paper in any resulting publications. | |
Melting Point |
68 °C | |
Record name | Avocadene | |
Source | Human Metabolome Database (HMDB) | |
URL | http://www.hmdb.ca/metabolites/HMDB0031042 | |
Description | The Human Metabolome Database (HMDB) is a freely available electronic database containing detailed information about small molecule metabolites found in the human body. | |
Explanation | HMDB is offered to the public as a freely available resource. Use and re-distribution of the data, in whole or in part, for commercial purposes requires explicit permission of the authors and explicit acknowledgment of the source material (HMDB) and the original publication (see the HMDB citing page). We ask that users who download significant portions of the database cite the HMDB paper in any resulting publications. | |
Retrosynthesis Analysis
AI-Powered Synthesis Planning: Our tool employs the Template_relevance Pistachio, Template_relevance Bkms_metabolic, Template_relevance Pistachio_ringbreaker, Template_relevance Reaxys, Template_relevance Reaxys_biocatalysis model, leveraging a vast database of chemical reactions to predict feasible synthetic routes.
One-Step Synthesis Focus: Specifically designed for one-step synthesis, it provides concise and direct routes for your target compounds, streamlining the synthesis process.
Accurate Predictions: Utilizing the extensive PISTACHIO, BKMS_METABOLIC, PISTACHIO_RINGBREAKER, REAXYS, REAXYS_BIOCATALYSIS database, our tool offers high-accuracy predictions, reflecting the latest in chemical research and data.
Strategy Settings
Precursor scoring | Relevance Heuristic |
---|---|
Min. plausibility | 0.01 |
Model | Template_relevance |
Template Set | Pistachio/Bkms_metabolic/Pistachio_ringbreaker/Reaxys/Reaxys_biocatalysis |
Top-N result to add to graph | 6 |
Feasible Synthetic Routes
Q1: What is the mechanism of action for Avocadene's anticancer activity?
A1: this compound, a polyhydroxylated fatty alcohol found in avocados, exhibits anticancer activity, particularly against leukemia cells. While this compound is often found in a 1:1 mixture with Avocadyne called Avocatin-B [], research suggests that this compound itself is a potent inhibitor of fatty acid oxidation (FAO) []. By suppressing FAO, this compound disrupts the energy metabolism of leukemia cells, leading to their elimination. Importantly, this compound appears to spare healthy blood cells [], indicating a potential therapeutic window.
Q2: How does this compound's structure contribute to its stability under food processing conditions?
A2: this compound, along with other acetogenins like persediene and persenones, demonstrates remarkable stability under various food processing conditions, including high temperatures (≤120°C), high hydrostatic pressure (HHP; 300–600 MPa), and varying pH levels []. This stability is attributed, in part, to the presence of a keto or trans-enone group at the C-4 position in the aliphatic chain of these molecules. This structural feature potentially contributes to antioxidant activity by supporting hydrogen donation to surrounding carbon atoms, thereby enhancing stability [].
Q3: What analytical methods are employed to quantify this compound in avocado fruit?
A3: While initial methods for detecting avocado seed polyhydroxylated fatty alcohols (PFAs) relied on NMR spectroscopy and GC-MS, these techniques lacked quantitative accuracy []. A more sensitive and accurate method utilizes LC-MS for the quantitation of this compound and Avocadyne in both avocado seed and pulp extracts []. This method offers a reliable linear response range and allows for precise quantification of these compounds.
Q4: Can this compound be used as an antimicrobial agent in food preservation?
A4: Research indicates that this compound, often in the form of enriched acetogenin extracts (EAE) from avocado seeds, exhibits potent antimicrobial activity, particularly against Gram-positive bacteria, including spore-forming species like Clostridium sporogenes, C. perfringens, Bacillus subtilis, and Alicyclobacillus acidocaldarius [, ]. Studies show that this compound-rich extracts effectively inhibit the growth of these bacteria, even surpassing the efficacy of commercial preservatives like Nisaplin® and Mirenat® in some cases [].
Q5: What is the potential of this compound for treating bacterial contamination in food?
A5: this compound, specifically an avocado seed extract commercially available as Avosafe®, has demonstrated promising results in controlling Listeria monocytogenes contamination in a refrigerated meat model system []. The extract effectively inhibited L. monocytogenes growth, reducing a 3-log inoculum to undetectable levels within 3 hours at 4°C []. This highlights the potential of this compound as a natural food preservative to enhance food safety.
Q6: Are there any known challenges or limitations associated with using this compound in practical applications?
A6: While this compound demonstrates potential in various applications, there are challenges to consider. For instance, although this compound remains stable under HHP processing, storage studies of a model food system revealed a gradual decline in its concentration over time (63% reduction at 25°C and 32% at 4°C over 42 days) []. This highlights the need for further research to optimize formulation strategies and storage conditions to maintain this compound's efficacy in food products.
Q7: How does the structure of this compound and its related compounds influence their mosquito larvicidal activity?
A7: While this specific question isn't directly addressed in the provided research abstracts, it is known that structural variations in related compounds like those found in unripe avocado fruit peel can significantly impact their biological activity, including mosquito larvicidal properties []. Further research is necessary to delve into the structure-activity relationships of this compound and its analogs specifically for their mosquito larvicidal potential.
Disclaimer and Information on In-Vitro Research Products
Please be aware that all articles and product information presented on BenchChem are intended solely for informational purposes. The products available for purchase on BenchChem are specifically designed for in-vitro studies, which are conducted outside of living organisms. In-vitro studies, derived from the Latin term "in glass," involve experiments performed in controlled laboratory settings using cells or tissues. It is important to note that these products are not categorized as medicines or drugs, and they have not received approval from the FDA for the prevention, treatment, or cure of any medical condition, ailment, or disease. We must emphasize that any form of bodily introduction of these products into humans or animals is strictly prohibited by law. It is essential to adhere to these guidelines to ensure compliance with legal and ethical standards in research and experimentation.