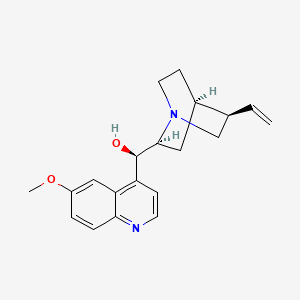
Quinoline alkaloid
- Click on QUICK INQUIRY to receive a quote from our team of experts.
- With the quality product at a COMPETITIVE price, you can focus more on your research.
Overview
Description
Quinoline alkaloids are naturally occurring chemical compounds derived from quinoline, a heterocyclic aromatic organic compound. These alkaloids are found in various plants, microorganisms, and animals. They are known for their diverse biological activities, including antiseptic, convulsive, and antineoplastic effects . Some well-known quinoline alkaloids include quinine, quinidine, cinchonine, and cinchonidine, which are primarily found in the bark of the Cinchona tree .
Preparation Methods
Synthetic Routes and Reaction Conditions: Several classical methods are used for the synthesis of quinoline and its derivatives:
Skraup Synthesis: This method involves the reaction of aniline with glycerol in the presence of sulfuric acid and an oxidizing agent like nitrobenzene.
Friedländer Synthesis: This involves the condensation of 2-aminobenzaldehyde with a carbonyl compound.
Doebner-Miller Reaction: This method uses aniline and α,β-unsaturated carbonyl compounds.
Industrial Production Methods: Industrial production of quinoline alkaloids often involves the extraction from natural sources, such as the bark of the Cinchona tree. The extraction process includes steps like direct crystallization, precipitation as insoluble salts, and extraction with polar solvents .
Types of Reactions:
Oxidation: Quinoline can undergo oxidation to form quinoline N-oxide.
Reduction: Reduction of quinoline can yield 1,2,3,4-tetrahydroquinoline.
Substitution: Quinoline can undergo electrophilic substitution reactions, such as nitration and sulfonation.
Common Reagents and Conditions:
Oxidation: Hydrogen peroxide or peracids are commonly used oxidizing agents.
Reduction: Catalytic hydrogenation using palladium or platinum catalysts.
Substitution: Sulfuric acid for sulfonation and nitric acid for nitration.
Major Products:
Oxidation: Quinoline N-oxide.
Reduction: 1,2,3,4-tetrahydroquinoline.
Substitution: 2-nitroquinoline and 2-sulfoquinoline.
Scientific Research Applications
Quinoline alkaloids have a wide range of applications in scientific research:
Chemistry: Used as scaffolds in the synthesis of various organic compounds.
Biology: Studied for their role in biological processes and interactions.
Medicine: Quinoline alkaloids like quinine and quinidine are used as antimalarial and antiarrhythmic agents.
Industry: Employed in the production of dyes, resins, and other industrial chemicals.
Mechanism of Action
Quinoline alkaloids can be compared with other similar compounds like isoquinoline alkaloids and acridine alkaloids:
Isoquinoline Alkaloids: These have a similar structure but differ in the position of the nitrogen atom in the ring.
Acridine Alkaloids: These contain an additional benzene ring fused to the quinoline structure.
Uniqueness: Quinoline alkaloids are unique due to their diverse biological activities and their presence in both natural and synthetic forms. Their ability to act as scaffolds for drug development makes them invaluable in medicinal chemistry .
Comparison with Similar Compounds
- Isoquinoline alkaloids (e.g., papaverine, berberine)
- Acridine alkaloids (e.g., acridine orange)
- Furoquinoline alkaloids (e.g., dictamnine)
- Pyranoquinoline alkaloids (e.g., skimmianine)
Properties
Molecular Formula |
C20H24N2O2 |
---|---|
Molecular Weight |
324.4 g/mol |
IUPAC Name |
(R)-[(2S,4R,5R)-5-ethenyl-1-azabicyclo[2.2.2]octan-2-yl]-(6-methoxyquinolin-4-yl)methanol |
InChI |
InChI=1S/C20H24N2O2/c1-3-13-12-22-9-7-14(13)10-19(22)20(23)16-6-8-21-18-5-4-15(24-2)11-17(16)18/h3-6,8,11,13-14,19-20,23H,1,7,9-10,12H2,2H3/t13-,14+,19-,20+/m0/s1 |
InChI Key |
LOUPRKONTZGTKE-FOEVPDMQSA-N |
Isomeric SMILES |
COC1=CC2=C(C=CN=C2C=C1)[C@H]([C@@H]3C[C@H]4CCN3C[C@@H]4C=C)O |
Canonical SMILES |
COC1=CC2=C(C=CN=C2C=C1)C(C3CC4CCN3CC4C=C)O |
Origin of Product |
United States |
Disclaimer and Information on In-Vitro Research Products
Please be aware that all articles and product information presented on BenchChem are intended solely for informational purposes. The products available for purchase on BenchChem are specifically designed for in-vitro studies, which are conducted outside of living organisms. In-vitro studies, derived from the Latin term "in glass," involve experiments performed in controlled laboratory settings using cells or tissues. It is important to note that these products are not categorized as medicines or drugs, and they have not received approval from the FDA for the prevention, treatment, or cure of any medical condition, ailment, or disease. We must emphasize that any form of bodily introduction of these products into humans or animals is strictly prohibited by law. It is essential to adhere to these guidelines to ensure compliance with legal and ethical standards in research and experimentation.