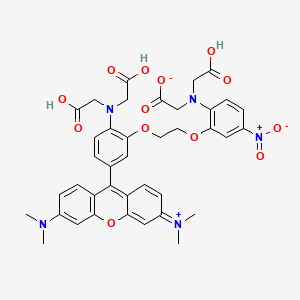
Rhod-5N (potassium salt)
- Click on QUICK INQUIRY to receive a quote from our team of experts.
- With the quality product at a COMPETITIVE price, you can focus more on your research.
Overview
Description
Rhod-5N (potassium salt) is a low-affinity fluorescent calcium indicator. It is particularly useful for studying compartments with high concentrations of calcium, such as the endoplasmic reticulum. Rhod-5N is essentially nonfluorescent in the absence of divalent cations but exhibits strong fluorescence enhancement upon binding calcium ions, with excitation and emission maxima at approximately 551 nm and 576 nm, respectively .
Preparation Methods
Synthetic Routes and Reaction Conditions: Rhod-5N is synthesized through a series of chemical reactions involving the parent compound Rhod-2The detailed synthetic route is proprietary and often involves multiple steps of organic synthesis, purification, and characterization .
Industrial Production Methods: Industrial production of Rhod-5N involves large-scale chemical synthesis under controlled conditions to ensure high purity and consistency. The process includes the use of high-quality reagents, solvents, and catalysts, followed by rigorous purification techniques such as chromatography and crystallization. The final product is then formulated into a stable, dry powder for distribution .
Chemical Reactions Analysis
Types of Reactions: Rhod-5N primarily undergoes complexation reactions with calcium ions. It does not typically participate in oxidation, reduction, or substitution reactions under normal conditions.
Common Reagents and Conditions:
Calcium ions (Ca²⁺): Rhod-5N binds to calcium ions in a buffered solution, resulting in a significant increase in fluorescence.
Buffer solutions: Commonly used buffers include HEPES and Hank’s Balanced Salt Solution (HHBS) to maintain physiological pH and ionic strength.
Major Products Formed: The primary product of the reaction between Rhod-5N and calcium ions is the fluorescent Rhod-5N-calcium complex, which is used for detecting and measuring calcium concentrations in various biological samples .
Scientific Research Applications
Rhod-5N is widely used in scientific research due to its unique properties as a calcium indicator. Some of its key applications include:
Chemistry: Used in fluorescence spectroscopy to study calcium dynamics in chemical reactions.
Biology: Employed in cell biology to measure intracellular calcium levels, particularly in organelles like the endoplasmic reticulum.
Medicine: Utilized in medical research to investigate calcium signaling pathways in various diseases, including neurodegenerative disorders and cardiovascular diseases.
Industry: Applied in the development of diagnostic assays and high-throughput screening methods for drug discovery
Mechanism of Action
Rhod-5N exerts its effects by binding to calcium ions, which induces a conformational change in the molecule, resulting in a significant increase in fluorescence. The binding affinity of Rhod-5N for calcium ions is characterized by a dissociation constant (Kd) of approximately 320 µM. This low affinity makes it suitable for detecting high concentrations of calcium in specific cellular compartments .
Comparison with Similar Compounds
Rhod-2: Higher affinity for calcium, used for detecting lower concentrations of calcium.
Fluo-3: Commonly used for general calcium measurements with moderate affinity.
Fluo-4: Similar to Fluo-3 but with improved fluorescence properties
Rhod-5N’s unique combination of low calcium affinity and high fluorescence enhancement makes it a valuable tool for specific applications where high calcium concentrations need to be measured accurately.
Properties
Molecular Formula |
C39H39N5O13 |
---|---|
Molecular Weight |
785.8 g/mol |
IUPAC Name |
2-[2-[2-[2-[bis(carboxymethyl)amino]-5-[3-(dimethylamino)-6-dimethylazaniumylidenexanthen-9-yl]phenoxy]ethoxy]-N-(carboxymethyl)-4-nitroanilino]acetate |
InChI |
InChI=1S/C39H39N5O13/c1-40(2)24-6-9-27-31(16-24)57-32-17-25(41(3)4)7-10-28(32)39(27)23-5-11-29(42(19-35(45)46)20-36(47)48)33(15-23)55-13-14-56-34-18-26(44(53)54)8-12-30(34)43(21-37(49)50)22-38(51)52/h5-12,15-18H,13-14,19-22H2,1-4H3,(H3-,45,46,47,48,49,50,51,52) |
InChI Key |
PVSMMTANYSSLPW-UHFFFAOYSA-N |
Canonical SMILES |
CN(C)C1=CC2=C(C=C1)C(=C3C=CC(=[N+](C)C)C=C3O2)C4=CC(=C(C=C4)N(CC(=O)O)CC(=O)O)OCCOC5=C(C=CC(=C5)[N+](=O)[O-])N(CC(=O)O)CC(=O)[O-] |
Origin of Product |
United States |
Disclaimer and Information on In-Vitro Research Products
Please be aware that all articles and product information presented on BenchChem are intended solely for informational purposes. The products available for purchase on BenchChem are specifically designed for in-vitro studies, which are conducted outside of living organisms. In-vitro studies, derived from the Latin term "in glass," involve experiments performed in controlled laboratory settings using cells or tissues. It is important to note that these products are not categorized as medicines or drugs, and they have not received approval from the FDA for the prevention, treatment, or cure of any medical condition, ailment, or disease. We must emphasize that any form of bodily introduction of these products into humans or animals is strictly prohibited by law. It is essential to adhere to these guidelines to ensure compliance with legal and ethical standards in research and experimentation.