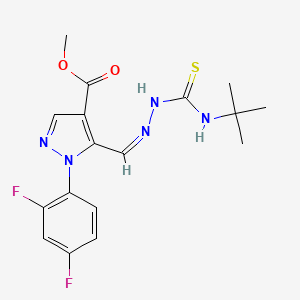
Methyl 1-(2,4-Difluoro-phenyl)-5-((4-tert-butyl-thiosemicarbazono)methyl)-1H-pyrazole-4-carboxylate
- Click on QUICK INQUIRY to receive a quote from our team of experts.
- With the quality product at a COMPETITIVE price, you can focus more on your research.
Overview
Description
ML194, also known as 1-(2,4-difluorophenyl)-5-[(2-[(1,1-dimethylethyl)amino]thioxomethyl]hydrazinylidene)methyl]-1H-pyrazole-4-carboxylic acid methyl ester, is a chemical compound with the empirical formula C17H19F2N5O2S and a molecular weight of 395.43 g/mol . It is a potent antagonist of the G protein-coupled receptor 35 (GPR35), which is expressed in various tissues including the nervous system, gastrointestinal tract, spleen, and immune cells .
Preparation Methods
Synthetic Routes and Reaction Conditions
The synthesis of ML194 involves multiple steps, starting with the preparation of the pyrazole core. The key steps include:
Formation of the pyrazole ring: This is typically achieved through the reaction of hydrazine derivatives with 1,3-diketones under acidic or basic conditions.
Introduction of the difluorophenyl group: This step involves the coupling of the pyrazole core with a difluorophenyl halide using palladium-catalyzed cross-coupling reactions.
Formation of the hydrazinylidene moiety: This is achieved by reacting the pyrazole derivative with a thiosemicarbazide under reflux conditions.
Esterification: The final step involves the esterification of the carboxylic acid group with methanol in the presence of a strong acid catalyst.
Industrial Production Methods
Industrial production of ML194 follows similar synthetic routes but on a larger scale. The process involves optimization of reaction conditions to maximize yield and purity, including the use of high-pressure reactors and continuous flow systems .
Chemical Reactions Analysis
Types of Reactions
ML194 undergoes various chemical reactions, including:
Oxidation: ML194 can be oxidized to form sulfoxides and sulfones using oxidizing agents such as hydrogen peroxide or m-chloroperbenzoic acid.
Reduction: The compound can be reduced to form hydrazine derivatives using reducing agents like lithium aluminum hydride.
Common Reagents and Conditions
Oxidation: Hydrogen peroxide, m-chloroperbenzoic acid.
Reduction: Lithium aluminum hydride, sodium borohydride.
Substitution: Amines, thiols, under basic or acidic conditions.
Major Products
Oxidation: Sulfoxides, sulfones.
Reduction: Hydrazine derivatives.
Substitution: Amino or thio-substituted pyrazole derivatives.
Scientific Research Applications
ML194 has a wide range of scientific research applications:
Chemistry: Used as a tool compound to study G protein-coupled receptor 35 (GPR35) signaling pathways.
Biology: Investigated for its role in modulating immune responses and gastrointestinal functions.
Medicine: Explored for potential therapeutic applications in pain management, hypertension, cancer, diabetes, and asthma.
Mechanism of Action
ML194 exerts its effects by antagonizing the G protein-coupled receptor 35 (GPR35). It binds to the receptor and inhibits its activation, thereby blocking downstream signaling pathways. This antagonistic action can modulate various physiological processes, including pain perception, immune responses, and gastrointestinal functions. ML194 is also known to rescue the cell-surface expression of mutant G protein-coupled receptor 35 (GPR35) receptors, likely acting as a pharmacological chaperone to alter the misfolded receptor structure .
Comparison with Similar Compounds
ML194 is compared with other G protein-coupled receptor 35 (GPR35) antagonists such as:
ML297: Another potent G protein-coupled receptor 35 (GPR35) antagonist with a different chemical structure.
SR1664: A selective G protein-coupled receptor 35 (GPR35) antagonist with distinct pharmacological properties.
ML194 is unique due to its high membrane permeability and potency, with an inhibitory concentration 50 (IC50) value of 160 nanomolar. It also has the ability to rescue misfolded G protein-coupled receptor 35 (GPR35) receptors, which is not observed with other antagonists .
Biological Activity
Methyl 1-(2,4-difluoro-phenyl)-5-((4-tert-butyl-thiosemicarbazono)methyl)-1H-pyrazole-4-carboxylate is a synthetic compound that has garnered attention for its potential biological activities, particularly in the fields of pharmacology and medicinal chemistry. This article explores its biological activity, focusing on its mechanisms, efficacy, and potential therapeutic applications based on diverse research findings.
Chemical Structure and Properties
The compound's structure is characterized by the presence of a pyrazole ring, difluorophenyl group, and a thiosemicarbazone moiety. These structural features are significant as they influence the compound's biological interactions.
Property | Value |
---|---|
Molecular Formula | C15H17F2N5O2S |
Molecular Weight | 363.39 g/mol |
CAS Number | 170985-86-1 |
Anticancer Activity
Research has indicated that compounds with similar structures exhibit anticancer properties. For instance, thiosemicarbazones are known for their ability to inhibit tumor growth and induce apoptosis in cancer cells. A study demonstrated that derivatives of thiosemicarbazone can effectively target multiple cancer cell lines through various mechanisms, including the inhibition of DNA synthesis and induction of cell cycle arrest .
Enzyme Inhibition
The compound may act as an inhibitor of specific enzymes involved in cancer progression. For example, it has been suggested that pyrazole derivatives can inhibit c-Jun N-terminal kinase (JNK), which plays a crucial role in cell proliferation and survival pathways .
Antimicrobial Activity
Preliminary studies have shown that thiosemicarbazones possess antimicrobial properties against various bacterial strains. This suggests that this compound may also exhibit similar activities, warranting further investigation into its potential as an antimicrobial agent.
The exact mechanism of action for this compound remains under investigation; however, it is hypothesized that its biological activity may involve:
- Inhibition of key signaling pathways: Targeting pathways such as JNK and p38 MAPK which are critical in cancer cell survival.
- Induction of oxidative stress: This can lead to apoptosis in cancer cells by disrupting cellular homeostasis.
- Interaction with DNA: Similar compounds have shown the ability to intercalate with DNA, leading to inhibition of replication.
Case Studies
Several studies have explored the biological activity of related compounds:
- Study on Thiosemicarbazones: A comprehensive study demonstrated that thiosemicarbazones exhibited significant cytotoxicity against various cancer cell lines, suggesting a promising avenue for drug development .
- Pyrazole Derivatives in Cancer Therapy: Research indicated that pyrazole derivatives could effectively inhibit tumor growth in vivo, supporting their potential as therapeutic agents against cancer .
- Antimicrobial Efficacy: A recent investigation into thiosemicarbazone derivatives found notable antibacterial activity against resistant strains of bacteria, highlighting their potential as novel antimicrobial agents .
Properties
Molecular Formula |
C17H19F2N5O2S |
---|---|
Molecular Weight |
395.4 g/mol |
IUPAC Name |
methyl 5-[(Z)-(tert-butylcarbamothioylhydrazinylidene)methyl]-1-(2,4-difluorophenyl)pyrazole-4-carboxylate |
InChI |
InChI=1S/C17H19F2N5O2S/c1-17(2,3)22-16(27)23-20-9-14-11(15(25)26-4)8-21-24(14)13-6-5-10(18)7-12(13)19/h5-9H,1-4H3,(H2,22,23,27)/b20-9- |
InChI Key |
CYNLZIBKERMMOA-UKWGHVSLSA-N |
Isomeric SMILES |
CC(C)(C)NC(=S)N/N=C\C1=C(C=NN1C2=C(C=C(C=C2)F)F)C(=O)OC |
Canonical SMILES |
CC(C)(C)NC(=S)NN=CC1=C(C=NN1C2=C(C=C(C=C2)F)F)C(=O)OC |
Origin of Product |
United States |
Disclaimer and Information on In-Vitro Research Products
Please be aware that all articles and product information presented on BenchChem are intended solely for informational purposes. The products available for purchase on BenchChem are specifically designed for in-vitro studies, which are conducted outside of living organisms. In-vitro studies, derived from the Latin term "in glass," involve experiments performed in controlled laboratory settings using cells or tissues. It is important to note that these products are not categorized as medicines or drugs, and they have not received approval from the FDA for the prevention, treatment, or cure of any medical condition, ailment, or disease. We must emphasize that any form of bodily introduction of these products into humans or animals is strictly prohibited by law. It is essential to adhere to these guidelines to ensure compliance with legal and ethical standards in research and experimentation.