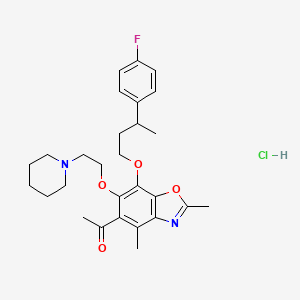
Kv1.3 Channel blocker 42
- Click on QUICK INQUIRY to receive a quote from our team of experts.
- With the quality product at a COMPETITIVE price, you can focus more on your research.
Overview
Description
Kv1.3 Channel Blocker 42 is a compound designed to inhibit the activity of the Kv1.3 potassium channel. This channel is a voltage-gated potassium channel that plays a crucial role in the activation and function of T lymphocytes, particularly effector memory T cells. The inhibition of Kv1.3 channels has been identified as a potential therapeutic strategy for treating autoimmune diseases such as multiple sclerosis, rheumatoid arthritis, and type 1 diabetes .
Preparation Methods
Synthetic Routes and Reaction Conditions: The synthesis of Kv1.3 Channel Blocker 42 typically involves the use of peptide synthesis techniques, as many Kv1.3 inhibitors are peptide-based. The synthesis can be carried out using solid-phase peptide synthesis (SPPS), which allows for the sequential addition of amino acids to a growing peptide chain. The reaction conditions often involve the use of protecting groups to prevent unwanted side reactions and the use of coupling reagents to facilitate the formation of peptide bonds .
Industrial Production Methods: Industrial production of this compound may involve large-scale peptide synthesis using automated peptide synthesizers. The process includes the purification of the synthesized peptide using high-performance liquid chromatography (HPLC) and the characterization of the final product using mass spectrometry and nuclear magnetic resonance (NMR) spectroscopy to ensure its purity and structural integrity .
Chemical Reactions Analysis
Types of Reactions: Kv1.3 Channel Blocker 42 primarily undergoes peptide bond formation reactions during its synthesis. Additionally, it may undergo oxidation and reduction reactions during the modification of its amino acid residues to enhance its stability and selectivity .
Common Reagents and Conditions:
Coupling Reagents: N,N’-Diisopropylcarbodiimide (DIC), O-Benzotriazole-N,N,N’,N’-tetramethyl-uronium-hexafluoro-phosphate (HBTU)
Protecting Groups: Fluorenylmethyloxycarbonyl (Fmoc), t-Butyloxycarbonyl (Boc)
Oxidizing Agents: Hydrogen peroxide, iodine
Reducing Agents: Dithiothreitol (DTT), tris(2-carboxyethyl)phosphine (TCEP)
Major Products: The major product formed from these reactions is the final peptide-based this compound, which is characterized by its high affinity and selectivity for the Kv1.3 potassium channel .
Scientific Research Applications
Kv1.3 Channel Blocker 42 has a wide range of scientific research applications, including:
Chemistry: Used as a tool to study the structure and function of potassium channels and their role in cellular physiology.
Biology: Employed in research to understand the role of Kv1.3 channels in immune cell function and signaling pathways.
Medicine: Investigated as a potential therapeutic agent for treating autoimmune diseases, neuroinflammatory disorders, and certain types of cancer.
Industry: Utilized in the development of diagnostic assays and screening platforms for identifying new Kv1.3 inhibitors
Mechanism of Action
Kv1.3 Channel Blocker 42 exerts its effects by binding to the Kv1.3 potassium channel and inhibiting its activity. This inhibition prevents the efflux of potassium ions, leading to the depolarization of the cell membrane and the subsequent inhibition of calcium influx. This disruption in ion homeostasis ultimately inhibits the activation and proliferation of effector memory T cells, which are implicated in the pathogenesis of autoimmune diseases .
Comparison with Similar Compounds
Kv1.3 Channel Blocker 42 can be compared with other similar compounds, such as:
ShK-186: A peptide derived from the sea anemone toxin ShK, known for its high affinity and selectivity for Kv1.3 channels.
HsTx1 [R14A]: An engineered scorpion toxin with enhanced selectivity for Kv1.3 channels.
Vm24: A natural scorpion toxin that inhibits Kv1.3 channels with high potency
Uniqueness: this compound is unique in its specific modifications that enhance its selectivity and stability compared to other Kv1.3 inhibitors. These modifications may include the incorporation of non-natural amino acids or the use of specific protecting groups during synthesis .
Biological Activity
Kv1.3 channel blockers, particularly compounds like Kv1.3 Channel Blocker 42, have garnered significant interest due to their potential therapeutic applications in autoimmune diseases and other inflammatory conditions. The Kv1.3 potassium channel is predominantly expressed in activated T cells and plays a critical role in regulating cellular activities such as proliferation, cytokine production, and calcium signaling. This article delves into the biological activity of this compound, examining its mechanisms of action, efficacy in various case studies, and its potential as a therapeutic agent.
The Kv1.3 channel facilitates potassium ion efflux, which is essential for maintaining membrane potential and regulating calcium influx during T cell activation. Inhibition of Kv1.3 disrupts this process, leading to decreased T cell activation and proliferation.
Key Mechanisms:
- Potassium Efflux Regulation: Kv1.3 channels allow K+ ions to exit the cell, which helps maintain a negative membrane potential necessary for calcium entry.
- Calcium Signaling Modulation: By blocking Kv1.3, the driving force for calcium influx through CRAC channels is reduced, inhibiting downstream signaling pathways that promote T cell activation and proliferation .
- Cytokine Production Inhibition: The blockade of Kv1.3 has been shown to decrease the production of pro-inflammatory cytokines, contributing to its immunosuppressive effects .
Efficacy in Case Studies
Numerous studies have evaluated the efficacy of this compound in various models of autoimmune diseases.
Table 1: Summary of Case Studies
Study | Condition | Model | Findings |
---|---|---|---|
Beeton et al., 2006 | Multiple Sclerosis | EAE Model | Demonstrated significant reduction in clinical symptoms with Kv1.3 blockade |
Wulff et al., 2009 | Rheumatoid Arthritis | Rat Model | Kv1.3 blockade led to decreased joint inflammation and damage |
Perez-Verdaguer et al., 2016 | Type 1 Diabetes | Mouse Model | Improved glycemic control with reduced T cell activity observed |
Research Findings
Research indicates that this compound exhibits high selectivity for the Kv1.3 channel over other potassium channels, which is crucial for minimizing side effects associated with broader potassium channel inhibition .
Selectivity and Potency:
- High Affinity: Studies have shown that this compound binds with high affinity to the Kv1.3 channel, effectively inhibiting its activity at low concentrations (sub-nanomolar levels) without significantly affecting other channels like Kv1.4 or Kv1.6 .
- Therapeutic Potential: Preclinical trials have indicated that this compound can ameliorate symptoms in models of autoimmune diseases without notable adverse effects .
Properties
Molecular Formula |
C28H36ClFN2O4 |
---|---|
Molecular Weight |
519.0 g/mol |
IUPAC Name |
1-[7-[3-(4-fluorophenyl)butoxy]-2,4-dimethyl-6-(2-piperidin-1-ylethoxy)-1,3-benzoxazol-5-yl]ethanone;hydrochloride |
InChI |
InChI=1S/C28H35FN2O4.ClH/c1-18(22-8-10-23(29)11-9-22)12-16-33-28-26(34-17-15-31-13-6-5-7-14-31)24(20(3)32)19(2)25-27(28)35-21(4)30-25;/h8-11,18H,5-7,12-17H2,1-4H3;1H |
InChI Key |
FHCGCBIHIRQNML-UHFFFAOYSA-N |
Canonical SMILES |
CC1=C(C(=C(C2=C1N=C(O2)C)OCCC(C)C3=CC=C(C=C3)F)OCCN4CCCCC4)C(=O)C.Cl |
Origin of Product |
United States |
Disclaimer and Information on In-Vitro Research Products
Please be aware that all articles and product information presented on BenchChem are intended solely for informational purposes. The products available for purchase on BenchChem are specifically designed for in-vitro studies, which are conducted outside of living organisms. In-vitro studies, derived from the Latin term "in glass," involve experiments performed in controlled laboratory settings using cells or tissues. It is important to note that these products are not categorized as medicines or drugs, and they have not received approval from the FDA for the prevention, treatment, or cure of any medical condition, ailment, or disease. We must emphasize that any form of bodily introduction of these products into humans or animals is strictly prohibited by law. It is essential to adhere to these guidelines to ensure compliance with legal and ethical standards in research and experimentation.