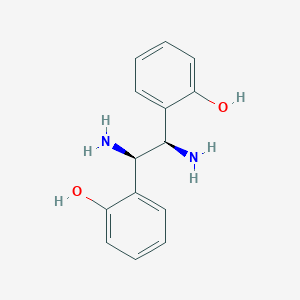
(1R,2R)-1,2-Bis(2-hydroxyphenyl)ethylenediamine
Overview
Description
(1R,2R)-1,2-Bis(2-hydroxyphenyl)ethylenediamine is a chiral diamine compound with the molecular formula C14H16N2O2. It is known for its stereoselective properties and is commonly used in the synthesis of enantiopure compounds. The compound is characterized by the presence of two hydroxyphenyl groups attached to an ethylenediamine backbone, making it a valuable intermediate in various chemical reactions.
Preparation Methods
Synthetic Routes and Reaction Conditions
The synthesis of (1R,2R)-1,2-Bis(2-hydroxyphenyl)ethylenediamine typically involves the reaction of 2-hydroxybenzaldehyde with (R,R)-1,2-diaminoethane. The reaction is carried out under controlled conditions to ensure the formation of the desired chiral product. The process involves the use of solvents such as ethanol or methanol and may require the presence of a catalyst to facilitate the reaction.
Industrial Production Methods
Industrial production of this compound involves large-scale synthesis using optimized reaction conditions to maximize yield and purity. The process may include steps such as crystallization and purification to obtain the final product in its purest form. The compound is often produced in powder form and is sensitive to air, requiring careful handling and storage.
Chemical Reactions Analysis
Types of Reactions
(1R,2R)-1,2-Bis(2-hydroxyphenyl)ethylenediamine undergoes various chemical reactions, including:
Oxidation: The compound can be oxidized to form quinone derivatives.
Reduction: Reduction reactions can lead to the formation of amine derivatives.
Substitution: The hydroxy groups can participate in substitution reactions to form ethers or esters.
Common Reagents and Conditions
Common reagents used in these reactions include oxidizing agents such as potassium permanganate, reducing agents like sodium borohydride, and alkylating agents for substitution reactions. The reactions are typically carried out under controlled temperatures and pH conditions to ensure the desired outcome.
Major Products
The major products formed from these reactions include quinone derivatives, amine derivatives, and various substituted compounds, depending on the specific reagents and conditions used.
Scientific Research Applications
(1R,2R)-1,2-Bis(2-hydroxyphenyl)ethylenediamine has a wide range of applications in scientific research, including:
Chemistry: It is used as a chiral ligand in asymmetric synthesis and catalysis.
Biology: The compound is employed in the study of enzyme mechanisms and protein interactions.
Medicine: It serves as a precursor for the synthesis of pharmaceutical compounds with potential therapeutic effects.
Industry: The compound is utilized in the production of advanced materials and fine chemicals.
Mechanism of Action
The mechanism of action of (1R,2R)-1,2-Bis(2-hydroxyphenyl)ethylenediamine involves its interaction with various molecular targets, including enzymes and receptors. The compound’s chiral nature allows it to selectively bind to specific sites, influencing biochemical pathways and processes. Its effects are mediated through the formation of stable complexes with metal ions, which can modulate the activity of target proteins and enzymes.
Comparison with Similar Compounds
Similar Compounds
(1S,2S)-1,2-Bis(2-hydroxyphenyl)ethylenediamine: The enantiomer of the compound with similar chemical properties but different stereochemistry.
1,2-Diphenylethylenediamine: A related compound with phenyl groups instead of hydroxyphenyl groups.
1,2-Diaminocyclohexane: A structurally similar compound with a cyclohexane backbone.
Uniqueness
(1R,2R)-1,2-Bis(2-hydroxyphenyl)ethylenediamine is unique due to its chiral nature and the presence of hydroxyphenyl groups, which enhance its reactivity and selectivity in chemical reactions. Its ability to form stable complexes with metal ions makes it a valuable tool in asymmetric synthesis and catalysis.
Properties
IUPAC Name |
2-[(1R,2R)-1,2-diamino-2-(2-hydroxyphenyl)ethyl]phenol | |
---|---|---|
Source | PubChem | |
URL | https://pubchem.ncbi.nlm.nih.gov | |
Description | Data deposited in or computed by PubChem | |
InChI |
InChI=1S/C14H16N2O2/c15-13(9-5-1-3-7-11(9)17)14(16)10-6-2-4-8-12(10)18/h1-8,13-14,17-18H,15-16H2/t13-,14-/m1/s1 | |
Source | PubChem | |
URL | https://pubchem.ncbi.nlm.nih.gov | |
Description | Data deposited in or computed by PubChem | |
InChI Key |
MRNPLGLZBUDMRE-ZIAGYGMSSA-N | |
Source | PubChem | |
URL | https://pubchem.ncbi.nlm.nih.gov | |
Description | Data deposited in or computed by PubChem | |
Canonical SMILES |
C1=CC=C(C(=C1)C(C(C2=CC=CC=C2O)N)N)O | |
Source | PubChem | |
URL | https://pubchem.ncbi.nlm.nih.gov | |
Description | Data deposited in or computed by PubChem | |
Isomeric SMILES |
C1=CC=C(C(=C1)[C@H]([C@@H](C2=CC=CC=C2O)N)N)O | |
Source | PubChem | |
URL | https://pubchem.ncbi.nlm.nih.gov | |
Description | Data deposited in or computed by PubChem | |
Molecular Formula |
C14H16N2O2 | |
Source | PubChem | |
URL | https://pubchem.ncbi.nlm.nih.gov | |
Description | Data deposited in or computed by PubChem | |
DSSTOX Substance ID |
DTXSID70584928 | |
Record name | 2,2'-[(1R,2R)-1,2-Diaminoethane-1,2-diyl]diphenol | |
Source | EPA DSSTox | |
URL | https://comptox.epa.gov/dashboard/DTXSID70584928 | |
Description | DSSTox provides a high quality public chemistry resource for supporting improved predictive toxicology. | |
Molecular Weight |
244.29 g/mol | |
Source | PubChem | |
URL | https://pubchem.ncbi.nlm.nih.gov | |
Description | Data deposited in or computed by PubChem | |
CAS No. |
870991-70-1 | |
Record name | 2,2'-[(1R,2R)-1,2-Diaminoethane-1,2-diyl]diphenol | |
Source | EPA DSSTox | |
URL | https://comptox.epa.gov/dashboard/DTXSID70584928 | |
Description | DSSTox provides a high quality public chemistry resource for supporting improved predictive toxicology. | |
Record name | (1R,2R)-1,2-Bis(2-hydroxyphenyl)ethylenediamine | |
Source | European Chemicals Agency (ECHA) | |
URL | https://echa.europa.eu/information-on-chemicals | |
Description | The European Chemicals Agency (ECHA) is an agency of the European Union which is the driving force among regulatory authorities in implementing the EU's groundbreaking chemicals legislation for the benefit of human health and the environment as well as for innovation and competitiveness. | |
Explanation | Use of the information, documents and data from the ECHA website is subject to the terms and conditions of this Legal Notice, and subject to other binding limitations provided for under applicable law, the information, documents and data made available on the ECHA website may be reproduced, distributed and/or used, totally or in part, for non-commercial purposes provided that ECHA is acknowledged as the source: "Source: European Chemicals Agency, http://echa.europa.eu/". Such acknowledgement must be included in each copy of the material. ECHA permits and encourages organisations and individuals to create links to the ECHA website under the following cumulative conditions: Links can only be made to webpages that provide a link to the Legal Notice page. | |
Retrosynthesis Analysis
AI-Powered Synthesis Planning: Our tool employs the Template_relevance Pistachio, Template_relevance Bkms_metabolic, Template_relevance Pistachio_ringbreaker, Template_relevance Reaxys, Template_relevance Reaxys_biocatalysis model, leveraging a vast database of chemical reactions to predict feasible synthetic routes.
One-Step Synthesis Focus: Specifically designed for one-step synthesis, it provides concise and direct routes for your target compounds, streamlining the synthesis process.
Accurate Predictions: Utilizing the extensive PISTACHIO, BKMS_METABOLIC, PISTACHIO_RINGBREAKER, REAXYS, REAXYS_BIOCATALYSIS database, our tool offers high-accuracy predictions, reflecting the latest in chemical research and data.
Strategy Settings
Precursor scoring | Relevance Heuristic |
---|---|
Min. plausibility | 0.01 |
Model | Template_relevance |
Template Set | Pistachio/Bkms_metabolic/Pistachio_ringbreaker/Reaxys/Reaxys_biocatalysis |
Top-N result to add to graph | 6 |
Feasible Synthetic Routes
Disclaimer and Information on In-Vitro Research Products
Please be aware that all articles and product information presented on BenchChem are intended solely for informational purposes. The products available for purchase on BenchChem are specifically designed for in-vitro studies, which are conducted outside of living organisms. In-vitro studies, derived from the Latin term "in glass," involve experiments performed in controlled laboratory settings using cells or tissues. It is important to note that these products are not categorized as medicines or drugs, and they have not received approval from the FDA for the prevention, treatment, or cure of any medical condition, ailment, or disease. We must emphasize that any form of bodily introduction of these products into humans or animals is strictly prohibited by law. It is essential to adhere to these guidelines to ensure compliance with legal and ethical standards in research and experimentation.