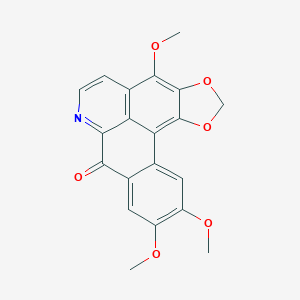
Thalicminine
Overview
Description
(3-Amino-propyl)-hexyl-phosphinic acid is a chemical compound that belongs to the class of phosphinic acids It is characterized by the presence of an amino group attached to a propyl chain, which is further connected to a hexyl chain and a phosphinic acid group
Preparation Methods
Synthetic Routes and Reaction Conditions
The synthesis of (3-Amino-propyl)-hexyl-phosphinic acid can be achieved through a phospha-Mannich reaction. This involves the reaction of hypophosphorous acid (H₃PO₂) with secondary amines and formaldehyde in wet acetic acid (AcOH). The reaction typically yields aminomethyl-H-phosphinic acids in nearly quantitative yields with minimal by-products . The reaction outcome is influenced by the basicity of the amines used, with more basic amines (pKa > 7-8) giving the desired products .
Industrial Production Methods
Industrial production methods for (3-Amino-propyl)-hexyl-phosphinic acid are not well-documented in the literature. the principles of large-scale organic synthesis, such as optimization of reaction conditions, use of continuous flow reactors, and purification techniques, can be applied to scale up the laboratory synthesis methods.
Chemical Reactions Analysis
Types of Reactions
(3-Amino-propyl)-hexyl-phosphinic acid can undergo various chemical reactions, including:
Oxidation: The phosphinic acid group can be oxidized to phosphonic acid.
Reduction: The amino group can be reduced to form secondary or tertiary amines.
Substitution: The amino group can participate in nucleophilic substitution reactions.
Common Reagents and Conditions
Oxidation: Common oxidizing agents such as hydrogen peroxide (H₂O₂) or potassium permanganate (KMnO₄) can be used.
Reduction: Reducing agents like lithium aluminum hydride (LiAlH₄) or sodium borohydride (NaBH₄) are typically employed.
Substitution: Nucleophilic substitution reactions can be carried out using alkyl halides or acyl chlorides under basic conditions.
Major Products Formed
Oxidation: Phosphonic acid derivatives.
Reduction: Secondary or tertiary amines.
Substitution: Alkylated or acylated derivatives of the original compound.
Scientific Research Applications
(3-Amino-propyl)-hexyl-phosphinic acid has several applications in scientific research:
Mechanism of Action
The mechanism of action of (3-Amino-propyl)-hexyl-phosphinic acid involves its interaction with molecular targets such as enzymes or receptors. The amino group can form hydrogen bonds or electrostatic interactions with the active sites of enzymes, leading to inhibition or modulation of their activity. The phosphinic acid group can mimic the transition state of certain enzymatic reactions, thereby acting as a competitive inhibitor .
Comparison with Similar Compounds
Similar Compounds
(3-Aminopropyl)triethoxysilane: An aminosilane used in surface functionalization and as a coupling agent.
Aminoalkylphosphonic acids: Compounds with similar structures but different functional groups, such as glyphosate.
Uniqueness
(3-Amino-propyl)-hexyl-phosphinic acid is unique due to its specific combination of an amino group, a propyl chain, a hexyl chain, and a phosphinic acid group. This unique structure imparts distinct chemical properties, making it suitable for specific applications in various fields.
Properties
IUPAC Name |
7,16,17-trimethoxy-3,5-dioxa-11-azapentacyclo[10.7.1.02,6.08,20.014,19]icosa-1,6,8(20),9,11,14,16,18-octaen-13-one | |
---|---|---|
Source | PubChem | |
URL | https://pubchem.ncbi.nlm.nih.gov | |
Description | Data deposited in or computed by PubChem | |
InChI |
InChI=1S/C20H15NO6/c1-23-12-6-10-11(7-13(12)24-2)17(22)16-14-9(4-5-21-16)18(25-3)20-19(15(10)14)26-8-27-20/h4-7H,8H2,1-3H3 | |
Source | PubChem | |
URL | https://pubchem.ncbi.nlm.nih.gov | |
Description | Data deposited in or computed by PubChem | |
InChI Key |
GOQUMRHFJDDYAU-UHFFFAOYSA-N | |
Source | PubChem | |
URL | https://pubchem.ncbi.nlm.nih.gov | |
Description | Data deposited in or computed by PubChem | |
Canonical SMILES |
COC1=C(C=C2C(=C1)C3=C4C(=C(C5=C3C(=NC=C5)C2=O)OC)OCO4)OC | |
Source | PubChem | |
URL | https://pubchem.ncbi.nlm.nih.gov | |
Description | Data deposited in or computed by PubChem | |
Molecular Formula |
C20H15NO6 | |
Source | PubChem | |
URL | https://pubchem.ncbi.nlm.nih.gov | |
Description | Data deposited in or computed by PubChem | |
DSSTOX Substance ID |
DTXSID60167704 | |
Record name | Thalicminine | |
Source | EPA DSSTox | |
URL | https://comptox.epa.gov/dashboard/DTXSID60167704 | |
Description | DSSTox provides a high quality public chemistry resource for supporting improved predictive toxicology. | |
Molecular Weight |
365.3 g/mol | |
Source | PubChem | |
URL | https://pubchem.ncbi.nlm.nih.gov | |
Description | Data deposited in or computed by PubChem | |
CAS No. |
16408-77-8 | |
Record name | Thalicminine | |
Source | ChemIDplus | |
URL | https://pubchem.ncbi.nlm.nih.gov/substance/?source=chemidplus&sourceid=0016408778 | |
Description | ChemIDplus is a free, web search system that provides access to the structure and nomenclature authority files used for the identification of chemical substances cited in National Library of Medicine (NLM) databases, including the TOXNET system. | |
Record name | Thalicminine | |
Source | EPA DSSTox | |
URL | https://comptox.epa.gov/dashboard/DTXSID60167704 | |
Description | DSSTox provides a high quality public chemistry resource for supporting improved predictive toxicology. | |
Retrosynthesis Analysis
AI-Powered Synthesis Planning: Our tool employs the Template_relevance Pistachio, Template_relevance Bkms_metabolic, Template_relevance Pistachio_ringbreaker, Template_relevance Reaxys, Template_relevance Reaxys_biocatalysis model, leveraging a vast database of chemical reactions to predict feasible synthetic routes.
One-Step Synthesis Focus: Specifically designed for one-step synthesis, it provides concise and direct routes for your target compounds, streamlining the synthesis process.
Accurate Predictions: Utilizing the extensive PISTACHIO, BKMS_METABOLIC, PISTACHIO_RINGBREAKER, REAXYS, REAXYS_BIOCATALYSIS database, our tool offers high-accuracy predictions, reflecting the latest in chemical research and data.
Strategy Settings
Precursor scoring | Relevance Heuristic |
---|---|
Min. plausibility | 0.01 |
Model | Template_relevance |
Template Set | Pistachio/Bkms_metabolic/Pistachio_ringbreaker/Reaxys/Reaxys_biocatalysis |
Top-N result to add to graph | 6 |
Feasible Synthetic Routes
Disclaimer and Information on In-Vitro Research Products
Please be aware that all articles and product information presented on BenchChem are intended solely for informational purposes. The products available for purchase on BenchChem are specifically designed for in-vitro studies, which are conducted outside of living organisms. In-vitro studies, derived from the Latin term "in glass," involve experiments performed in controlled laboratory settings using cells or tissues. It is important to note that these products are not categorized as medicines or drugs, and they have not received approval from the FDA for the prevention, treatment, or cure of any medical condition, ailment, or disease. We must emphasize that any form of bodily introduction of these products into humans or animals is strictly prohibited by law. It is essential to adhere to these guidelines to ensure compliance with legal and ethical standards in research and experimentation.